A permanent magnet attracts. Application of magnets
magnetism magnetism Why is a magnet called a magnet?
Indeed, why do we call it that? But as soon as they did not try to name a magnet before! The ancient Greeks - "special stone", "that stone", and also "Herculean stone", either because of its strength, or because this stone was mined near the city of Heraclea in Lydia. The Greeks had another name - "siderite", in translation - "diamond". But don't think it's because of the hardness or beauty of the magnet. It’s just that the diamond itself was called siderite due to the purely “iron” brilliance in its raw form, the Greeks also called soft iron. The Greek name siderite was due to the "tendency" of the magnet to iron, and perhaps due to the fact that the magnet was originally mined in the mines of iron ores.
Later, the British, French, Spaniards, and then the Greeks themselves were deceived by this duality of the name and based their modern nicknames on the magnet diamond. This is how the French "aimant", the Spanish "piedramant", the English "adamant" and the modern Greek "adamas" turned out. True, they say that the French did not mean diamond - adamas, but the ancient Chinese name for the magnet “chu-shi” or “nitshi-chi”, which means “loving stone”. And in French, “aimant” - (pronounced “eman”) is “loving”.
I must say that the entire Ancient East endowed the magnet with the ability to love iron. If it attracts, then it loves. And therefore, almost all the Eastern names of the magnet originate from this property - for example, the Sanskrit “thumbaka”.
The Italians called the magnet "calamita", and this word began to be used in Romania, Bosnia and in the same Greece.
Rice. 328. Natural magnets: a - in "helmets"; b - in a frame with a magic symbol
The old German name for the magnet is also known: "sigelstein" - "printed stone". It probably occurs because of the custom, widespread in antiquity, to carve various magical figures and symbols on natural magnets (Fig. 328), and such stones could already be used as seals. The great scientist Isaac Newton even wore a ring, where a natural magnet of extraordinary strength was present as a precious stone. It is possible that the scientist also sealed wax seals on letters and documents with them ... And, finally, the Egyptians called the magnet the Ora bone. By the name Or they meant the property of the Sun to rise and set. In other words, Or is one of the deities ancient egypt, whose bone was thought to be a magnet.
That's how many names this wonderful stone had, but we still call it a magnet.
The ancient Greek philosopher Plato reports that the poet Euripides gave this name to the stone. But for no reason at all, you can’t invent a word. According to a legend described by the ancient historian Pliny, borrowed from even more ancient sources, a shepherd from the island of Crete named Magnis or Magness noticed that his iron-shod sandals, as well as a stick with an iron tip, stick to black stones, which were lying in abundance under your feet. The shepherd turned the stick over with the “unshod” end and made sure that the wood was not attracted by strange stones that do not recognize any other materials than iron. Apparently, the shepherd took a few of these stones from Mount Ido, where he was herding sheep, home and struck the imagination of the neighbors. From the name of the shepherd came the name "magnet".
There is another explanation for the word "magnet" - by the name of the province of Magnesia in Ionia near the Meander River. The inhabitants of this province were called magnets. The Roman scientist and poet Titus Lucretius Carus, in his poem "On the Nature of Things", paying much attention to magnets, directly indicates:
“This stone was called by the Greeks by the name of the deposit by the magnet, since it was found within the magnets.” The German name for a magnet, "magnet", seems to have something to do with it.
Now this province is called Manissa, and magnetic stones are still found there. The local Mount Sipil, rich in natural magnets, is often struck by lightning strikes, just like Magnitnaya Mountain in the Urals. It has long been noticed that a magnet attracts lightning.
For over 2,000 years people have been using the properties of a magnet. And, perhaps, the Hercules stone was used in the compass first of all.
What is a south indicator?
Fast forward to Ancient China. The Chinese historian Su Matzen, having studied the ancient chronicles, left us an interesting story about the events that took place in ancient times.
More than 4 thousand years ago, Emperor Huang Ti attacked the enemy from the rear with an army in thick fog and won. He was helped in this, according to the chronicle, by figures mounted on wagons with an outstretched hand, always pointing to the south (Fig. 329).

Or another legend. In the caravans that in ancient times went through the Gobi desert to the west, there was a special, white camel. This camel carried an unusual load - an earthen vessel with water placed in a wooden protective cage. A raft made of cork tree bark floated on the water, on which an oblong piece of Chu-shi stone or a steel needle rubbed with this stone was fixed. The edges of the vessel were painted in colors that symbolically denoted parts of the world: red - south, black - north, green - east, white - west. (And now they often paint the south pole of a magnet red, and the north pole black or blue. Is it because red is warm and blue is cold?) The magnet on the cork, easily turning on the water, always indicated the direction "south - north. A vessel with water and a magnet was perhaps the very first compass used by the caravan in the desert.
Chinese compasses have survived to our time, however, in a rather damaged form; they are kept in museums. One of these compasses, dating back to the X century. n. e., outwardly surprisingly similar to a modern wooden spoon placed on a tray (Fig. 330). The handle of this “spoon” is an oblong magnet, and the bottom of the spoon is a good ball bearing, easily rotating on a “tray”, on which divisions are applied, allowing you to determine the countries of the world, even the southwest, southeast, northwest and northwest. East.
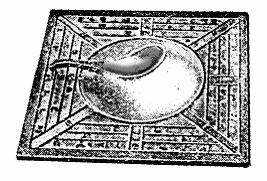
In the XI-XII centuries. The south indicator has already become known as "chi nan ting", or "arrow pointing south", which is closer to the modern concept of "magnetic compass needle". It is also known that they were able to prepare artificial magnets from a steel needle rubbed with a magnetic stone. This magnetized needle was used as a "south pointer" or ancient compass.
It is sometimes believed that the compass came from China through India to the Arabs, and from the Arabs to the Europeans, and this happened in the 12th century. But, probably, the idea of a compass did not penetrate from China to Europe, and this device was invented there independently. The inventor of the compass in Europe is the Italian Flavio Gioia, a native of the city of Amalfi. In Naples, they even erected a monument to him, and in 1902 they solemnly celebrated the 600th anniversary of this invention. True, there were mentions of a “European” compass by the monk Alban Nequem in 1187 and the poet Guyot of Provence in 1206.
However, before the invention of Joya, the European compass, although it had an arrow, did not have a rotary dial with divisions, which made it very difficult to use it. Yes, and this device was called not a compass, but a tremor, a magnetic turntable and even a frog. Then appeared modern name device - from the Italian "compassare", which means "to measure in steps." And Joy's merit was at least the fact that he supplied the device with the missing rotary dial with divisions, giving it modern look. With the help of the compass invented by Joya, all the great geographical discoveries were made.
Are magnetic temptations strong?
On the author's desk lies a thick book with the profile of a sage embossed on the cover. It was published in 1600 in London. The person who wrote it did more to study magnets than anyone else. His name is William (William) Gilbert (1544-1603). The English poet Dryden said of him:
Gilbert will live as long as
until the magnet stops attracting...
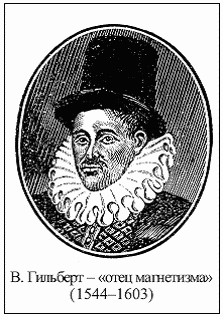
Galileo, after reading Gilbert's book, declared him "great to such an extent that it causes envy."
In the famous Oxford University, for a long time hung a portrait of Gilbert, depicted in full growth, in a doctoral robe, holding in his hand a magnetic model of the globe - a terrelu. Above the left shoulder of the scientist are written the words: "The first prospector of magnetic forces Gilbert." Contemporaries and descendants called Gilbert the father of magnetism.
And all these words are gratitude to the scientist for his famous book "On the magnet, magnetic bodies and the big magnet - the Earth", which he wrote for 18 years.
Gilbert collected numerous parables and superstitions about the magnet, created by ancient scientists, "deceivers and storytellers," as he called them.
Here is what Hilbert himself writes:
“For example, the suspicion expressed about the magnet, whether it was created insidiously by evil demons ... Or that the magnet unlocks any locks and shutters and benefits thieves with its smoke and fumes, as if this stone arose for theft. Or as if iron, attracted by a magnet and placed on a scale, adds nothing to the weight of the magnet, as if the weight of the iron were absorbed by the strength of the stone. Or as if in India there are some sea rocks abounding with a magnet, which extract all the nails from the ships that have stuck to them ... and in the construction of them you have to use wooden nails so that they do not vomit. Or they say that there is another mountain in Ethiopia, which is generated by the stone feamed, which cannot bear iron, throws it out and pushes it away from itself.
“With such nonsense and fairy tales,” Hilbert remarks emotionally, “vulgar philosophers themselves amuse themselves and feed those readers who are thirsty to know the mysterious and the ignoramuses who amuse themselves with absurdities.”
It is interesting that not only in antiquity, but even today, various extraordinary phenomena were associated and continue to be associated with a magnet. What just did not try to achieve with the help of magnets! And fly to the moon, and build a "perpetual motion machine", and create a new type of weapon. There is something in common in all these attempts, which it would be appropriate to call magnetic temptations.
It all started again with China. According to a legend that arose many centuries ago, Emperor Shi Huangdi ordered to revet the gate with a magnetic stone, opening the way to his palace. And if a warrior in iron armor tried to pass through these gates, he would freeze in place, attracted by a magnet. Moreover, if an intruder with a hidden weapon tried to get through this gate, then it escaped from him and stuck to the magnetic vault, just like nails torn out of the ship by a “magnetic mountain”. Most likely, this is fiction, since natural magnets could not have such power.
It is characteristic that the idea of wresting weapons from the hands of the enemy with magnets has survived millennia and even reached the century before last. In the novel "On Two Planets" the writer of the XIX century. Kurt Lasswitz describes the battle of earthlings with martians. The Earth cavalrymen boldly marched against the Martian air machines and seemed to force them into the air to fly away. But the cunning Martians unfolded between their air machines something in the form of a huge blanket that covered the battlefield from above. This veil turned out to be ... a huge magnet of extraordinary power. The rest happened in the same way as in the Chinese magnetic gates. In the blink of an eye, the air was filled with a thick cloud of spears, sabers and carbines, flying up with thunder and crackling towards the magnetic blanket, to which they stuck.
Or a fantastic project appeared to save ships from enemy cannonballs. The idea was to install powerful magnets on the ship towards the enemy, covered with thick armor. The enemy cores were supposed to be attracted by a nearby magnet, turn in its direction and break against strong armor. The rest of the ship could have been left unprotected.
In principle, everything was true, except that even the most powerful of magnets cannot act at a great distance. Imagine that we have a magnet that can attract 10 tons of iron at a distance of 1 cm. This is a very strong magnet. So, if we move the useful object another 1 cm, then the force of attraction will drop 8 times! At a distance of 1 m, the force of attraction will drop by a factor of 1,000,000, and there can be no talk of any attraction of the nuclei.
But in the last century, they still did not know how to calculate the strength of magnets, and such a magnet-armor was nevertheless built in 1887. This magnet attracted a steel plate so that a force of 10 tons was needed to tear it off. Four 120-kilogram cores hung one after another on the pole of a magnet. But 2 m from the magnet, people who had steel objects in their pockets barely felt the effect of the magnet. There was nothing to think about the attraction of the enemy nuclei. True, such a magnet acted on the compass needle for 10 km.
And this served as the invention of the so-called magnetic mines. It is known that steel bodies are spontaneously magnetized under the influence of terrestrial magnetism. This is especially true for long objects - bridges, ships. So, such a magnetized ship, sailing over a mine installed at the bottom, affects a device such as a magnetic needle located in a mine. The mine floats up and explodes near the ship.
Such magnetic mines can be easily neutralized. A plane with a powerful magnet on board is launched along the ship's route. This magnet causes the mine to float and explode when the plane itself is already far ahead. And besides, the ship can be "demagnetized" - to create such a magnetic field with additional magnets, which is equal, but opposite to the magnetic field of the ship. This was often done during the Great Patriotic War, in particular, the future great physicist I. V. Kurchatov was engaged in this in the Crimea.
Is a magnetic "perpetual motion machine" possible?
Numerous "perpetuum mobile" designs have been associated with magnets, which have proved difficult to debunk.
In chronological order, it looks like this. Even in the XIII century. Pierre Peregrine de Maricourt, a medieval magnet researcher, argued that if a magnetic stone is turned into a regular ball and its poles are directed exactly along the axis of the world, then such a ball will spin and spin forever.
De Maricourt himself did not make such an experiment, although he had magnetic balls, and he did other experiments with them. Apparently, he believed that he himself had not made the ball accurately enough or had directed its poles not along the axis of the world. But he persistently advised readers to make and test a magnetic perpetual motion machine, adding: “If it comes out, you will enjoy it, if not, blame your little art!”
The same author has a description of another "perpetual motion machine" - a gear wheel with teeth made of steel and silver through one. If you bring a magnet to this wheel, de Maricourt argued, the wheel will begin to rotate. Here de Maricourt was very close to building, though not eternal, but at least a thermal engine, which at that time would undoubtedly be considered "eternal". But more about that later, but for now, about the "real" "perpetual motion machines".
There were a great many lovers of making magnetic "perpetual motion machines". English Bishop John Wilkens in the 17th century. even received official confirmation of his invention of a "perpetual motion machine", but the latter did not work from this. On fig. 331 shows the principle of its operation. According to the author, a steel ball, attracted by a magnet, rises along the top inclined plane, but before reaching the magnet, it falls into the hole and rolls along the lower tray. Having rolled down, he again falls on his former path and so forever continues his movement.
In fact, everything turned out differently. If the magnet was strong, then the ball did not fall into the hole, but jumped over it and stuck to the magnet. If the magnet was weak, then the ball stopped halfway on the lower tray, or did not leave the bottom point at all. And here is the “perpetual motion machine”, which the author himself built in childhood, and was very surprised when he did not work.
A steel ball was placed in a round plastic box, planted on a spoke, like a wheel on an axle. A magnet had to be brought in front, and the box-wheel had to spin on a spoke (Fig. 332). Still: the ball was attracted by a magnet, rose along the wall of the box, like a squirrel in a wheel, as the same squirrel began, falling down, to turn the wheel. However, the wheel did not want to turn. As it turned out, the ball rose under the influence of a magnet, pressing against the wall of the box, and was not going to fall down.
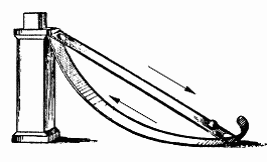

But there are also real magnetic motors, which at first glance look like eternal ones.
Even Hilbert himself noticed that if iron is heated strongly, then it completely ceases to be attracted by a magnet. Now the temperature at which iron, steel or alloys lose their magnetic properties is called the Curie point, after the physicist Pierre Curie, who explained this phenomenon. If these magnetic properties were not lost, then the red-hot blanks in the forges could be carried by magnets, which is very tempting.
But this property made it possible to create the so-called magnetic mill, or carousel. We hang a wooden disk on a thread or put it on a steel needle like a compass needle. Then we will stick several knitting needles into it and put the pole of a strong magnet on the side (Fig. 333). Why not de Maricourt's cogwheel? Of course, like that wheel, our mill will not rotate until we heat the spoke adjacent to the magnet in the flame of the burner and impart rotation with a slight push. The heated needle is no longer attracted to the magnet, and the next one tends to it until it hits the flame of the burner. In the meantime, the heated spoke will go a full circle, it will cool down and be attracted again by the magnet.
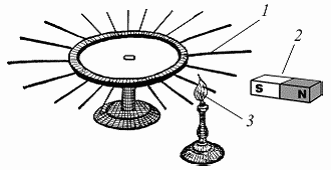
Why not a perpetual motion machine? And the fact that it takes the energy of the burner to rotate it. Therefore, this engine is not eternal, but thermal, in principle the same as in cars and diesel locomotives.
Working on the same principle, a magnetic swing is easy to build yourself. We hang a small iron object on a wire to the top of the swing rack. The easiest way is to take a long piece of iron wire and roll its end into a small ball. Then we put a magnet on a small stand, one pole pointing sideways. We will move the stand with the magnet to the suspended iron lump until it is attracted to the magnet.
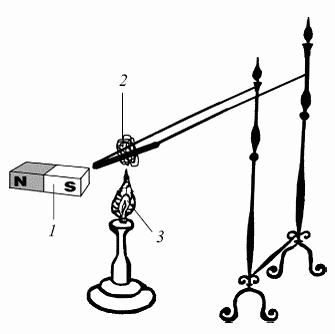
Now let's substitute a spirit lamp, a candle or another burner under the swing so that the lump is above the flame itself (Fig. 334). After some time, having heated up to the Curie point, it will fall away from the magnet. Swinging in the air, it will cool down again and will again be attracted to the pole of the magnet. It will turn out an interesting swing that will swing until we remove the burner.
A ball rolled from wire is good for the experiment in that it both heats up and cools faster than, for example, a solid steel ball. Therefore, such a swing will swing more often than with a ball on a thread.
In practice, this principle is sometimes used for automatic hardening of small steel objects, such as needles. Cold needles hang, attracted by a magnet, and heat up. As soon as they are heated to the Curie point, they cease to be attracted and fall into the quenching bath.
Ordinary iron has a rather high Curie point: 753 °C, but now alloys have been obtained for which the Curie point is not much higher than room temperature. Heated by solar heat, such a material, especially dark-colored, is no longer magnetic. And in the shade, the magnetic properties are restored, and the material can again be attracted. For example, the metal gadolinium has a Curie point of only 20 °C.
The inventor and journalist A. Presnyakov created an engine based on this principle that continuously pumps water in a hot desert. The sun fully provides it with its energy. Even a trolley has been built, automatically moving towards the sun and even an electric lamp (Fig. 335). Such engines, operating on clean and free energy from the Sun, are very promising, especially in the exploration of the Moon and other planets. Why not the "perpetual motion machines" that de Maricourt dreamed of?
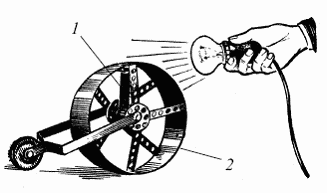
Does Mohammed's coffin fly?
The story of the coffin of the prophet Mohammed, flying, or, more precisely, levitating, in a magnetic cave, has been haunting the minds of scientists for more than one century.
Apparently, the great Gilbert himself, the father of magnetism, was the first to tell the world about the strange floating coffin of Mohammed. In his book “On Magnets…”, published in 1600, he refers to a certain Mattiol, who “... gives a story about the chapel of Mohammed with a vault of magnets and writes that this extraordinary phenomenon (an iron chest hanging in the air) strikes the crowd like some kind of divine miracle.
Back in 1574, the Italian Giolamo Fracostro wrote in his book On Sympathy: power to pull iron up as much as iron rushes down. Iron seems to be strengthened in the air.
Hilbert debunks this claim by Fracostro. “This is absurd,” writes Hilbert, “since the closer magnetic force is always the more powerful. Due to the fact that the force of the magnet does not lift iron much from the earth, it must be constantly excited by the magnet (if there are no obstacles) and stick to it.
That is, there can be no question of any stable position of a piece of iron suspended in a magnetic field.
Interestingly, even before Gilbert, the Italian Porta was convinced of the impossibility of this. In his book with the exotic title " natural magic”, published in 1589, Porta, desperate to make the magnet hover under a piece of iron, writes: “But I say that this can be done, because I have now done it to keep the magnet on an almost invisible thread so that it hangs in the air: only so that a small thread is tied to it below, so that it cannot rise higher.
Rice. 336. Experience with an electromagnet
This interesting experiment is often done by schoolchildren, forcing a needle to hang on a thread “upside down” under a magnet. This spectacular experience was repeated by a German company producing electromagnets. Chained to the floor was a heavy iron ball, bursting into the sky. A worker even rises along the chain to the ball - and the ball continues to hang (Fig. 336). But the trick is that a lifting electromagnet is mounted above the ball, which attracts it so strongly that the ball does not fall, despite the large air gap between it and the magnet. Meanwhile, the gap greatly reduces lifting force magnet - even a piece of paper placed between the poles of a school horseshoe magnet and an attracted piece of iron reduces the force of attraction by half.
Naturally, Gilbert denied the rumors about the free floating of the chest in the chapel of Mohammed. But in 1647, in the book of the German Jesuit scientist Athanasius Kircher “On the Magnet”, a coffin floating in the air already appears - “The coffin of Mohammed is held in the air by magnetic force.”
But the most amazing thing is that the great scientist, mathematician and physicist Leonhard Euler also believed in the possibility of free magnetic suspension of iron objects! In his book “Letters on Various Physical and Philosophical Matters, Written to a Certain German Princess of the Academy of Sciences by a Member, Astronomer and Professor”, which went through over forty editions, translated into ten foreign languages, says: “They say that the tomb of Mohammed is held by the power of some magnet; it does not seem impossible, because there are artfully made magnets that lift up to a hundred pounds. It turns out that Euler did not doubt even the possibility of creating magnets with a large lifting force. The question of whether the lifted body will linger at the point of balance or fall to one side or the other, like a pencil placed on a point, was left unattended by the scientist.
Euler's book was written in 1774, and only in 1842 did Professor S. Earnshaw publish the article "The Nature of Molecular Forces" in the "Notes of the Cambridge University", where he proved that a ferromagnetic body located in a field permanent magnets, cannot be in a state of stable equilibrium. That is, Earnshaw did with the help of mathematics what Hilbert expressed in words - he imposed a ban on the free floating of magnets and the metals attracted by them. And by no combination of magnets and pieces of iron it is possible to suspend either one or the other so that they do not touch any other bodies.
As for the notorious tomb of Mohammed, was it really impossible to go there, to the city of Medina on the Arabian Peninsula, and visit the legendary tomb - Hijra, as the Muslims call it, which is located in the Haram mosque, and see for yourself whether this coffin is floating in the air?
No, it turns out it wasn't easy at all. Many travelers paid with their lives for their curiosity. Fanatical pilgrims immediately killed any "infidel" who entered Medina. At the beginning of the XIX century. a certain Burkgardt went to Medina for this purpose, who was fortunate enough to see the Haram mosque. For a lot of money, he managed to look into a small window and look at the tomb. But in the window he saw only ... a curtain. The riddle has not been solved.
Finally, in 1853, a brave English officer, Richard Burton, went to Medina on the instructions of the London Geographical Society. He was dressed in a pilgrim's robe and well aware of how to behave in appropriate situations so that he would not be recognized as a European. Several times Barton was on the verge of exposure, but as a result everything went well, and he was the first among Europeans who managed to penetrate the holy of holies of Muslims - the Haram Mosque, inspect the famous tomb and return home alive.
And soon, in 1854, Barton's book "Description of the Journey to Mecca and Medina" was published, where the tomb of Mohammed was described in detail. It was an ordinary crypt, in which there were not one, but three coffins - Mohammed and his two relatives. These coffins were by no means made of iron (or crystal, according to other legends), but ordinary, wooden, although richly decorated. And of course, there was no question of a huge magnet supporting them.
As the Russian journal Biblioteka dlya chteniya wrote about this, “thus stories similar to the fables of the Thousand and One Nights disappear as soon as there is an opportunity to verify them by observation.” Gold words!
Which magnetism is longitudinal and which is transverse?
In 1939, the German scientist Dr. W. Braunbeck performed a real miracle by suspending tiny bodies in a constant magnetic field. Moreover, these bodies hovered in the air, not touching anything, just like the mythical coffin of Mohammed (Fig. 337). But it is necessary to talk about these bodies especially, since they were made of their substances, which until the 20th century. considered to be non-magnetic.
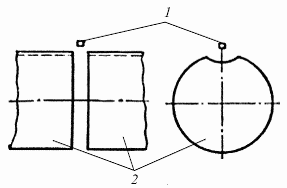
We know that a magnet attracts iron objects. In addition to iron, metals close to it are also attracted - nickel and cobalt. Such metals are called ferromagnets. If these metals are heated to the Curie point, then they cease to be attracted to the magnet - this was already known to Hilbert. But to be precise, they continue to attract, only hundreds of thousands of times weaker. These metals become paramagnetic. For example, gadolinium metal becomes a ferromagnet only at temperatures below 16 ° C, and above it is paramagnetic. The Curie point for him comes at room temperature. There are a lot of paramagnets. These metals are magnesium, calcium, aluminum, chromium, manganese, oxygen gas and many others.
But it turns out that there are many more other substances - diamagnets, which are repelled by a magnet. True, this repulsion of diamagnets is very weak and difficult to notice.
Back in 1778, the little-known scientist Anton Brugmans put a piece of bismuth metal into a small paper boat, put it on the water and brought a magnet to it. And contrary to the common sense of that time, the boat began to sail away from the magnet. This result was so unusual that the scientists did not even check it, but simply did not believe Brugmans. Too great was the authority of Hilbert, who claimed that there could be no bodies repelled by a magnet.
It's amazing how much trouble the authority of a scientist can entail! Even in simple things, where common sense simply tells you to check the opinion of an authority, people prefer to believe this opinion and not check it.
So, Aristotle claimed that the fly has four legs, and women have more teeth in their mouths than men. And almost 1,500 years after Aristotle, no one bothered to catch a fly and count the number of its legs or count the number of teeth in his wife's mouth. And what is the advice of ancient scientists on how to reduce the strength of magnets with the help of garlic or diamonds! Hilbert's enormous authority was needed to refute this well-established but fundamentally wrong opinion. But the same Gilbert writes: “Pliny, an outstanding person ... copied from others a fairy tale that has become well known in modern times, thanks to frequent retellings: in India, near the Indus River, there are two mountains; the nature of one, consisting of a magnet, is such that it retains all iron; the other, consisting of feamed, repels iron. So, if there are iron nails in the shoes, then there is no way to tear off the soles from one of these mountains, and there is no way to step on the other. Albertus Magnus writes that in his time a magnet was found which attracted iron on one side and repelled it on the other side.
The legend of a certain stone feamed, borrowed from Pliny, is often found in medieval books. The same Pliny can be read: “They say that there is another mountain in Ethiopia, and not far from the Zimiri (magnetic mountain) named above, which is generated by the feamed stone, which cannot bear iron, throwing it out and pushing it away from itself.”
Perhaps the ancients noticed that some substances, including graphite, the strongest diamagnetic and widespread material in nature, are repelled by a magnet. Who could prevent anyone else in ancient times from carrying out the simple experiment of Brugmans by placing a piece of graphite on a floating cork or plank? This would be the beginning of the doctrine of diamagnets, which formed the basis of the legends about feamedes.
The result of Hilbert's authoritative statement was that no one believed Brugmans. True, later his experiments were repeated by the French scientist Henri Becquerel (grandfather of the famous Henri Becquerel, who discovered the radioactivity of uranium) and naturally came to the same result. Little by little, scientists leaned into the idea that bismuth is still repelled by a magnet, but this is an exception to the rule. The opinion that only three metals - iron, nickel and cobalt are attracted to a magnet, and all other substances are indifferent to it, dominated science until 1845. Because it was in this year that the great English scientist Michael Faraday (1791-1867) established, that there are no substances in nature that are completely indifferent to a magnet. Faraday believed that natural forces are one and magnetic properties are inherent in all substances existing in nature.
In order to reveal even an insignificant ability of bodies to be attracted or repelled by a magnet, Faraday suspended these bodies on a thin long thread between the poles of a powerful electromagnet. The longer the thread was, the less force was required to deflect - attract or repel - the body. Indeed, when a suspended body deviates, it moves in an arc and slightly rises. The Earth's gravity tends to return the body to its original, lowest position and prevents deflection. But the longer the thread, the less curvature of the arc and the less effort is required to deflect it. No matter how heavy the load, even hundreds of tons, if it is suspended on a long rope, the assembly workers easily deflect it with their hands, accurately aiming at the landing site.
With this method, Faraday tested thousands of substances and made sure that absolutely all the studied bodies react to a magnetic field in a different way, to a different extent. Several metals and alloys - ferromagnets - are strongly attracted by a magnet. A greater number of substances, which Faraday called paramagnets, are attracted, and a huge number of substances - all other substances, except for ferromagnets and paramagnets - are repelled by a magnet. Faraday called them diamagnets.
The words "paramagnets" and "diamagnets" are distinguished by the prefixes "para" and "dia". These prefixes in Greek mean "along" and "across". If you take rods from a paramagnetic and a diamagnetic, hang them on a thread or put them on a needle, and bring them into the field between the two poles of a magnet, then they will behave differently. A paramagnetic, like a ferromagnetic, rod, the ends of which are attracted to the poles of the magnet, will be located along lines of force fields - from pole to pole (Fig. 338, a). A diamagnetic rod, the ends of which, when approaching the pole of the magnet, acquire the same polarity, will tend to take such a position that the ends are away from any poles of the magnet, that is, perpendicular to the magnetic field lines (Fig. 338, b). Hence the name of these magnets. The number of diamagnets is enormous, it is certainly greater than the list that Faraday compiled on the basis of his experiments: “Iodine, wax, gum arabic, ivory, dried lamb, dried beef, fresh beef, fresh blood, dried blood, bread, Chinese ink, Berlin porcelain, silkworm, charcoal… this list is very long. Even man himself is also a diamagnet.”
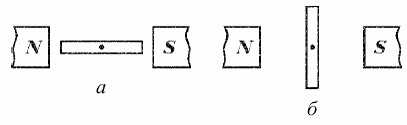
“If it were possible to hang a person on a sufficiently sensitive suspension,” wrote Faraday, “and place him in a magnetic field, then he would be located across the lines of force, since all the substances of which he is composed, including blood, have this property.”
To emphasize how comprehensive diamagnetism is, it is said that all substances in nature are diamagnets; as an exception to the rule, there are paramagnets, and very rarely - ferromagnets. But all the time it was believed that only these "rare" ferromagnets have magnetic properties!
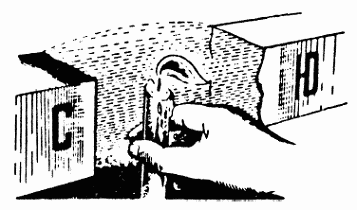
But after all, Hilbert could not help but know that the flame of a candle is repelled from the pole of a magnet, is pushed out of the magnetic field, since the products of combustion are diamagnetic (Fig. 339). In addition, Hilbert often placed pieces of iron and magnets on a floating cork and observed their attraction, repulsion of the poles of the same name, and the orientation of the magnet to the poles of the Earth. What did it cost him, suspecting the repulsion of some substances in the composition of the flame from the magnet, to place soot, soot, or even a piece of candle on a cork raft and bring a strong magnet to it? This should have been done at least in order to be convinced of the impossibility of the feameds. Thousands and thousands of various experiments were carried out by Hilbert, but he did not begin to carry out this experiment, because he did not see the point in it, being convinced in advance that there could be no substances repelled by a magnet. But in vain!
Returning to the man-made "coffin of Mohammed" by Dr. V. Brownback, it should be noted that it was diamagnets suspended in a magnetic field - bismuth and graphite. The first weighed 8 milligrams, and the second weighed 75. The magnetic field strength between the poles of the magnet was 23,000 oersteds, which is a lot.
What kind of suspension is magnetic?
In 1939, the German scientist W. Braunbeck proved that, in principle, it is possible to hang the coffin of Mohammed. For this, it would be best to make it from graphite, although wood is suitable, it is already diamagnetic. But it is difficult to carry out this idea: to suspend such massive objects, a magnetic field of monstrous intensity of a huge volume is needed.
Dr. Brownback used an electromagnet for his experiments, otherwise he would not have been able to obtain such a high magnetic field strength with the help of permanent magnets of that time. But the electromagnet required a constant supply of current. From an energy point of view, it turned out even insulting - a voracious electromagnet capable of lifting tons raises milligrams.
In 1956, the Dutch scientist A. Boerdik implemented a non-contact suspension, and without power consumption. Bourdick's experience is as follows: a cylindrical permanent magnet is vertically installed above a hemisphere of a strong diamagnet - graphite. And in the gap between them is placed a small, weighing about 2 milligrams, a magnet in the form of a microscopic washer the size of a pinhead. The magnet is magnetized so that one end of it is the North Pole, the other is the South Pole.
And the magnet hangs in this gap (Fig. 340).
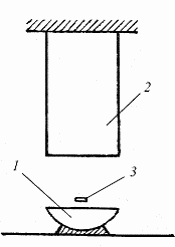
1 - graphite hemisphere; 2 - large magnet; 3 - small magnet
Why is this happening? On the one hand, diamagnetic graphite tries to push away the magnetic washer. But the puck, even if the forces of the diamagnet were enough for this, would still fall off or turn on its side. The diamagnet did not count on this - it simply provides all possible assistance to the magnet in order to only tear off the magnetic puck from its surface. In addition, the magnet centers this washer, prevents it from turning on its side or on edge.
The forces of magnetic attraction are not enough to tear off an object from any surface and pull it towards you with lightning speed. They are only enough to slightly raise the washer with the help of a diamagnet, after which the force of diamagnetic repulsion of graphite will sharply decrease. So the magnetic puck hangs, being unable to either fall on the graphite or be attracted to the pole of the magnet. Needless to say, a floating magnet and a large magnet face each other with opposite poles.
Whose suspension was better - Brownback or Boerdik? Hard to say. Here comes to mind a very accurate comparison of these suspensions with a helicopter and a balloon. Which one is better to use for lifting a load? The helicopter, holding the load, constantly expends energy to rotate the propeller - it's like a Brownback suspension. The balloon does not consume energy for this, but it is much larger than a helicopter, and if it is the same size, then it lifts much less cargo - this is the Boerdik suspension.
But what if we use some combination of a helicopter and a balloon, i.e. build a magnetic airship? Such an attempt was made by the German scientist E. Shteingrover, and his magnetic suspension was literally Hercules in comparison with the Braunbeck and Boerdik suspensions. The Steingrover suspension, using the properties of both ferromagnets and diamagnets, made it possible to hang a disk in a precision electrical appliance weighing as much as 50 g! This is 1,000 times more than before.
The main weight in the Steingrover's suspension is "held" by a permanent ring-shaped magnet, which centers the small cylindrical magnets and pulls them up. But since such a position is unstable (remember Earnshaw's prohibition!), then the axis of the disk, on which these rods are mounted, must immediately jump up or down. The inventor designed it in such a way that it would tend to go down a little. But here the axis is supported by a diamagnetic bearing in the form of a graphite ring, repelled by a strong permanent magnet. And the repulsion is small - only 0.04 N, but this is enough to make the magnetic suspension stable (Fig. 341).

1 - permanent ring-shaped magnet; 2 – cylindrical magnets; 3 - suspended disk; 4 - graphite ring; 5 - bottom magnet
These are the tricks that had to be resorted to in order to hang a part weighing only 50 g without any contact with other objects!
More, it seemed, could only be dreamed of. However, several years ago, according to newspaper reports, scientists from the University of Nottingham in England placed a live frog in a magnetic field so powerful that it, like an ordinary diamagnet, began to float in the air!
The experiments began with solid diamagnets - bismuth, antimony, continued on liquid ones - acetone, propanol and reached living plants and animals - frogs and fish. And in the fall of 1997, again, according to newspaper reports, the world's first levitation attraction for animals opened in the Japanese city of Osaka. Pets manage to soar on
height up to 17 m. They say that they really like to fly. Apparently, a strong magnetic field does not harm them, at least momentarily.
They do not dare to place people in such a strong magnetic field - studies on the effects of such fields on living organisms have not yet been completed. The magnetic fields used to levitate living beings are unusually strong - thousands of times stronger than those created by ordinary permanent magnets and many orders of magnitude stronger than the field of terrestrial magnetism where these creatures used to live.
Well, these coffins of Mohammed magnetic fields will not harm, and therefore its levitation is not excluded at all!
Is the suspension "hot"?
Now let's talk about the hot suspension. This, of course, is not about heat. If we simply heat the load or the winding holding it in suspension, we will achieve little. The effect of heating here is obtained, as it were, by itself; it's a side effect.
The history of hot suspension goes back to the 90s of the XIX century, when the American inventor Elihu Thompson demonstrated his famous experience. The essence of the experience was this. The inventor put an aluminum ring on a cylindrical electromagnet with a core of iron wires, and then connected an alternating current of a sufficiently high frequency to the winding. At the same time, the ring soared up above the core and flew away to the side (Fig. 342). What force threw the ring up?
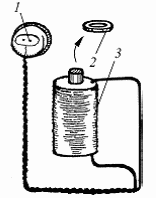
1 - plug; 2 - aluminum ring; 3 - electromagnet
When the direction of the current in the winding of the electromagnet changes, its polarity changes, and therefore, the magnetic induction in the core changes sharply both in magnitude and in sign. If such an electromagnet is placed near a closed conductor winding, an induction (induced) current will appear in it. He, in turn, creates his own magnetic field, opposing the magnetic field of the electromagnet.
And the aluminum ring is the same winding, only from one turn.
And the electromagnet seeks to quickly push the magnetic field of the ring out of its own, and with it the ring itself. What happened in the experiment of E. Thompson.
In this case, alternating current is not so necessary at all. An inductive current can be induced by moving a conductor near the pole of a magnet. For example, in electric meters, an aluminum disk, rotating between the poles of a strong magnet, is decelerated due to induction (eddy) currents that occur in the disk.
The disk experience can be presented as reminiscent of E. Thompson's experiment. We unwind a copper or aluminum top and bring a sufficiently strong magnet close to it from the side (Fig. 343, a). The top will immediately move away from the magnet and will stubbornly evade it, no matter where we bring the magnet from. Moreover, the repulsion of the magnetic fields of the magnet and the induced current can significantly exceed the force of attraction by the magnet of a ferromagnetic body, at least the same top. If we spin rather strongly not an aluminum, but an iron top, then at a high frequency of rotation it will repel the magnet, and at a low frequency it will be attracted to it. It has been noticed that a metal flywheel rotating over a magnet, as it were, loses weight (Fig. 343, b).
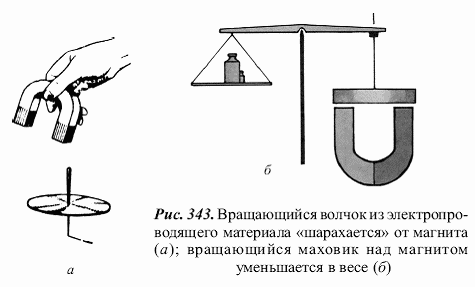
And now about the side effect of heating suspended bodies in such a suspension.
At the New York World's Fair in 1939, this amazing hot pan suspension, then just a toy, was shown in the pavilion "Wonders of Technology". The frequency of the current was only 60 Hz - the usual industrial frequency of the current in the USA (in our country - 50 Hz), the diameter of the pan was 300 mm. Although the author was not at this exhibition, since he was born just in the year of its opening, he saw such an installation and even made sure that the weighed frying pan heats up by induction current. The author saw such an installation in the 50s. 20th century in our country in ... a circus. Yes, yes, it was successfully demonstrated in the circus by an artist named Sokol, and the aluminum frying pan was the most ordinary, only without a handle, and the electromagnet was built into the top of ... the refrigerator.
The effect was amazing: in a frying pan hanging in the air above the refrigerator (Fig. 344), fried eggs were fried and even treated to the audience! And then this toy began to work in technology, and it turned out to be very promising. Now the future of metallurgy of special alloys is associated with it. The fact is that when melting some metals and alloys, their contact with the crucible in which they are usually melted is unacceptable, therefore, melting in a suspended state turned out to be a real find in the production of such alloys, for example, ultra-pure or aggressive alloys that react with the crucible.
An installation for melting metals in suspension appeared for the first time in 1952 and looked a little different than the toy described. The windings are made in the form of an upper flat and a lower funnel-shaped coil, fed by an audio frequency current of about 10,000 Hz. A piece of metal was placed on the lower coil, which needed to be melted, and the current was turned on. The metal floated up between the coils and began to heat up (Fig. 345). Having melted, it took the form of a top and descended. The molten metal could be cooled by reducing the current, and then, by further reducing the current, it could be placed in a solid state on the lower coil.
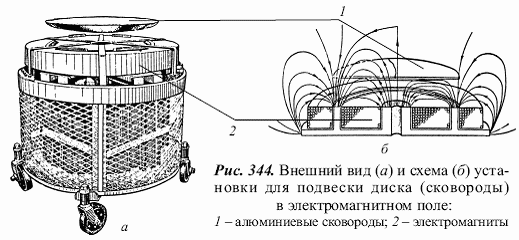
This is how aluminum, titanium, silver, gold, indium, tin and other metals were melted, and in an atmosphere of inert gases, hydrogen and in a vacuum. Such melting is especially useful for titanium, which easily reacts with the material of the crucible in the molten state.
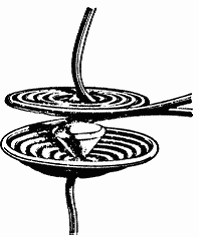
What kind of trains are flying?
Flying trains are considered to be the transport of the 21st century; work on them is being carried out in all technically developed countries. And it all started in 1910, when the Belgian E. Bachelet, a simple worker-fitter who did not receive any special education, built the world's first flying train model and tested it. E. Bachelet worked hard to implement his idea for almost 20 years. Of course, for the transport of passengers, his model was small, but still made a stunning impression on his contemporaries. Still - a 50-kilogram cigar-shaped car of a flying train accelerated to an unheard-of speed at that time - over 500 km / h!
The Bachelet magnetic road was a chain of metal posts with coils mounted on their tops. While there was no current in these coils, the car lay motionless on them. But after turning on the current, the trailer rose above the coils and hung in the air. Now even a child could move him. But there was no need to push this trailer - it accelerated itself, by the same magnetic field on which it was suspended.
The flying car of E. Bachelet caused a sensation all over the world, it was called a miracle of the 20th century. In France, they decided to use E. Bachelet trailers instead of the then popular pneumatic city mail, in England they were going to build a full-scale model of the E. Bachelet road with large wagons. But then the work stopped, and once sensational projects were forgotten.
Almost simultaneously with Bachelet - in 1911 - Professor of the Tomsk Technological Institute B. Weinberg develops a much more economical suspension for a flying train. In contrast to E. Bachelet, Weinberg proposed not to push the road and the cars away from each other, which is fraught with enormous energy costs, but to attract them to each other with ordinary electromagnets. Of course, the road must be located on top of the car in order to compensate for the gravity of the train with its attraction.
However, any magnet, including an electric one, if, by attracting, has moved the body from its place, then it will certainly attract it to itself until it touches. Fortunately, the electromagnet can be turned off in time, and the body will stop at any predetermined distance from it.
But Weinberg's flying train was more cunning. The iron wagon was originally located not exactly under the electromagnet, but somewhat behind it. At the same time, electromagnets were suspended on the "ceiling" of the road along its entire length with a certain interval between them.
Letting current into the first electromagnet, we caused both the rise of the iron trailer and its advancement towards the magnet. But a moment before the trailer was supposed to touch the electromagnet and stick to it, the current was turned off, and the trailer, continuing to fly forward due to the speed it had gained, began to decrease in height. Then the next electromagnet was turned on, and the trailer, falling into its magnetic field, again rose up, increasing the forward speed. So, along a wave-like trajectory, the trailer "ran" from magnet to magnet without touching them (Fig. 346).
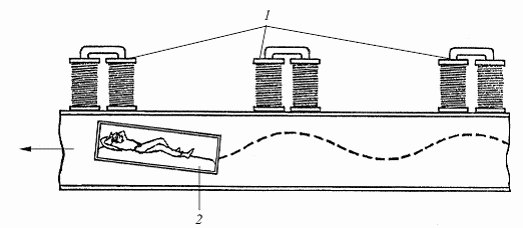
Professor Weinberg turned out to be more far-sighted than Bachelet in another way. Knowing the great air resistance when moving any body, including a car, at high speeds, the inventor placed his car in a non-magnetic copper pipe, from which he pumped out air. And if Bachelet, in order to reduce air resistance, gave his trailer a cigar-shaped streamlined shape, then for B. Weinberg the streamlining of the trailer was useless. Since there was practically no air inside the pipe, there was also no resistance - the trailer had the shape of an ordinary cylinder. Electromagnets were attached to the upper part of the pipe, which accelerated B. Weinberg's trailer to a speed of 800 km / h! Only shells of large-caliber short-barreled guns - mortars and mortars - flew at such a speed. Of course, it would be even more economical to use strong permanent magnets instead of electromagnets, but the trouble is that they cannot be turned off! The train would inevitably be attracted to the ceiling and stick to it.
Here it is just right to remember that science and technology many times again and again turned to the old, it would seem, already obsolete solutions. No wonder they say that the new is the well-forgotten old. All this is fully applicable to the suspension of flying trains. If you don't want the magnet to stick to the magnet, reverse the polarity of one of them and they will repel (Fig. 347)!
Rice. 347. Repulsion of like poles of magnets is the principle of magnetic suspension
So the experts on the suspension of flying trains came again to the idea of Bachelet, but instead of electromagnets alternating current used conventional permanent magnets. The road over which the train was to be suspended was paved with magnets so that they were facing upwards with the same poles. The bottom of the wagon was also covered with magnets, also facing downwards with the same poles, but in such a way that the wagon was repelled from the road (Fig. 348).
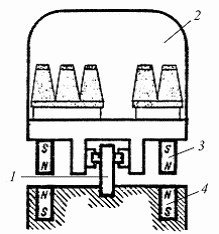
1 - slipper; 2 - wagon; 3 – car magnet; 4 - road magnet
At least two conditions must be met here: the magnets must be strong enough to lift the car off the road, and in addition, the car must not fall on its side - because permanent magnet suspension, as we know from Earnshaw's ban, is unstable.
It is believed that at speeds above 500 km / h it is already dangerous to use conventional wheels. Special wheels made of ultra-strong and lightweight materials allow for short-term doubling of speed, such as on record racing rocket cars. But these are very unreliable wheels, and it is because of their breakdowns that accidents most often occur.
Meanwhile, for testing missiles on the ground, sleds are often used, sliding along guide rails. They withstand speeds several times greater than the speed of sound, however, with large energy losses - after all, you have to bear the brunt of the tested devices. The slippers, which protect the car on the magnetic track from falling sideways, practically do not carry any loads, so the energy consumption and wear in them are negligible.
Let's return to the question - will the permanent magnets have enough strength to keep the car above the road. In Hilbert's time it would hardly have been possible to build such a road. But since then, the possibilities of permanent magnets have grown significantly.
At the beginning of the XX century. chromium, tungsten and cobalt steels began to be used for permanent magnets, and in the 30s. – special magnetic alloys, which make it possible to obtain very strong magnets. Moreover, it is not at all necessary that the components of these alloys be ferromagnets. It seems paradoxical, but, for example, the Heusler alloy, consisting of two paramagnets (manganese and aluminum) and one diamagnet (copper), is a strong ferromagnet. Or an amazing alloy - silmanal. It also does not contain any ferromagnet: manganese, silver and aluminum. Silmanal gives very strong permanent magnets, and unlike most of them, it is not fragile. Silmanal magnets can be processed on machine tools, rolled into a tape, and made into wire.
But the most practical magnetic alloy is alnico, which consists of aluminium, nickel and cobalt, and many permanent magnets are still made from it. In the 50s. 20th century cheap and light magnets based on barium ferrites, a cheap and very common material in Russia, were obtained.
True, there are magnets - champions in their properties, but they are very expensive. For example, an alloy of platinum with cobalt will make it possible to obtain a magnet capable of lifting an iron load 2,000 times its own weight.
However, the recently appeared permanent magnets made of rare earth materials samarium, neodymium and praseodymium in their alloy with cobalt and iron are more promising. Magnets made from rare earth elements, such as samarium-cobalt, having a strength no less than platinum-cobalt magnets, are much cheaper than them. Modern prices for these magnets are only a few times higher than for ordinary ones, but how many times stronger are they!
But let's not focus on these promising magnets for now. Even cheap ferrites, with which one of the existing magnetic roads is paved, with a gap between the magnets of 10 mm, make it possible to obtain a lifting force of 12.3 kN per square meter of paved track area. The mass of the magnets themselves, for example, for a 100-seat car, designed for a speed of 450 km/h, amounted to 18% of the total weight of the car. The advantage of such a magnetic road is its simplicity and the absence of energy costs for suspending the train.
If we talk about the prospects, a speed greater than 500 km / h, only air resistance prevents us from developing. There is only one way out of this situation - the one that Professor Weinberg used. By placing a flying train in a pipe or tunnel, from which the air is pumped out, you can get not only supersonic, but also cosmic speed. And the vacuum in the tube should not be afraid: today's pressurized aircraft fly in an atmosphere that differs little in rarefaction from that in a tube for a maglev train. The promising project of the Planetran road, which should connect the east and west coasts of the United States, provides for a maglev train in a vacuum tube tunnel. The speed of the train is 22,500 km/h, which is almost equal to the first cosmic speed!
It is convenient to move so quickly, especially in such big country like Russia. Note that on no aircraft other than space rocket, such a speed cannot be developed. And in a vacuum tube - please. And no fuel consumption with an oxidizer is needed - the train in the pipe will be accelerated by a traveling magnetic field, as in electric motors, which will be discussed later. And a huge kinetic energy, which this train will accumulate, can be taken from it in the same way, only in the braking mode. Just like in elevators: when lifting a load potential energy accumulates, and during the descent, it is given back to the network through the electric motor.
Excuse me, but such a train could serve as an excellent energy storage device on a global scale! After all, each kilogram of mass moving at a speed of 8 km / s accumulates energy of 32 MJ, or almost 10 kWh. This is an unheard of high specific energy storage capacity. And with a train mass, for example, 10 6 kg, which is an average indicator, it will accumulate almost 10 million kWh of energy. The accumulated energy of this order could significantly improve the energy system, not only major country but also the whole world. In one part of the earth it is day, in another it is night. The accumulated energy could be supplied to that part of the world where it is most needed. If we focus on solar energy, then its excess in that part of the world where it is light should also be accumulated based on cloudy weather or at night. In the developed world, the cost of electricity at night is much less than during the day, and a storage device could balance this cost.
One trouble - the train came to the final destination, and like it or not - allocate all the accumulated energy to stop! But this can be avoided if such a high-speed road is closed in a ring. Calculations show that for this it is not at all necessary to stretch the road across the entire globe, although this would be the best. The author calculated that a ring road the size of the Moscow Ring Road (100 km long) would be quite enough, at least for the needs of the whole country. At the same time, the train itself must be closed in a ring, and the dimensions of the “cars” along the cross section can be only 1 × 1 m. Naturally, the pipe where such energy-accumulating trains will “fly”, as in the Planetran system, is vacuum, and the suspension is magnetic. The author designed the project of such a "super-accumulator" as a Russian invention, maybe someday in the future it will come in handy. Again, the Russians will be the first here.
And if we do not talk about global projects, then magnetic suspension can already help today as bearings for large flywheels (again, drives!), Turbines and similar heavy rotating parts. What's wrong with conventional bearings? Yes, because, firstly, they require lubrication and care, which, for example, is difficult in a vacuum. Secondly, their durability leaves much to be desired. And thirdly, - energy losses for rotation, which, by the way, go to the destruction of the same bearings.
Magnetic suspension, based on ordinary permanent magnets, centered on miniature, almost unloaded bearings (so as not to lose Earnshaw stability!), Is able to provide the following "record" performance:
- durability - decades with almost no maintenance;
– low energy losses for rotation;
– high speeds, inaccessible to conventional bearings.
A diagram of such a magnetic suspension is shown in Fig. 349. In order to minimize losses and mass of magnets, they are grouped around the center in a column or battery. A number of tricks that make up the invention were also used, namely, suspension body parts, which were previously just ballast, were used as active elements. In addition, an optimal - gentle - dependence of the lifting force on vertical displacements has been achieved. That is, if the lifting force of the suspension is 15 kN, then it will not change when the gap between the magnets changes - from an assembly error or thermal expansion.
Such a suspension, which has a record low ratio of the mass of the magnet to the mass of the suspended load (less than 0.5%), was developed by the author for one of the German energy companies and manufactured at a specialized Moscow enterprise. Load capacity 15 kN (flywheel weight - 1.5 t); magnets based on the composition "iron - neodymium - boron" are quite inexpensive.
At present, such strong permanent magnets and such “smart” suspension systems have been created that in the near future we should expect a wide use of magnetic bearings in technology instead of conventional ones.

1 - flywheel; 2 - fixed magnet; 3 - moving magnet
Pour me half a liter of... a magnet!
For a long time, people have tried to make magnetic fluids by agitating fine powders of ferromagnetic material in water, oil, and other liquids. But nothing good came of it, the suspension of the powder in the liquid - the suspension - disintegrated, and the powder settled: the particles of the material turned out to be too large and heavy.
But in the 60s. 20th century the ferrite powder was so well ground in a ball mill that, being poured into a mixture of kerosene and oleic acid, it ceased to precipitate. Mankind has received a liquid magnet.
What is the matter here? It turns out that the powder particles were already so small that the thermal (Brownian) movement of the molecules did not allow them to settle, and a colloidal solution was obtained, which is known to us as egg white, stationery glue and many similar substances. Translated from Latin, such a solution is called glue, glue-like. Most adhesives - carpentry, silicate, etc. - are also colloidal solutions.
It turned out that the magnetic fluid has new, very interesting properties. First of all, a magnetic fluid is not a ferromagnet, but the strongest paramagnet - a superparamagnet. If you pour a magnetic fluid into a glass and bring a magnet from below, then it forms an antinode, which at first glance is completely improbable for liquids - a hillock, almost hard to the touch (Fig. 350). If you bring the magnet to the side, then the liquid will climb the wall and can rise as high as you like behind the magnet. If it is poured over the surface of the water, then a magnet lowered into the water can quickly collect it on the pole of the magnet. It is bad if it is a permanent magnet, it will not be so easy to “tear off” the tenacious liquid from the magnet. If the magnetic fluid is poured in a trickle from one glass to another, then it is very easy to steal it by bringing a magnet to the side of the trickle.
Whoever sees this viscous, heavy, dark brown liquid for the first time does not believe that liquids can behave this way in the presence of magnets. It seems to be a clever trick.
Now many useful applications have been invented for magnetic fluids: for sealing shafts and pistons, for “eternal” lubrication, for collecting oil spilled on water, for mineral processing, for treating and diagnosing many diseases, and even for direct conversion of thermal energy into mechanical energy.
Let's talk about the most interesting and promising applications of magnetic fluid for technology. Here you have to introduce surfactants and apply other tricks so that the liquid turns out to be stable and does not deteriorate, i.e., does not coagulate (coagulate like milk), does not dry out, does not exfoliate, etc.

Finally, the magnetic fluid is ready. Where can it be used?
It is most widely used for sealing - sealing gaps between moving parts of machines. Most often it is necessary to seal rotating shafts. When the shaft is ferromagnetic (for example, steel), then a ring magnet with two washers is put on the shaft with a gap, the gaps of which with the shaft - one or both - are filled with magnetic fluid. She immediately rushes into the gap, where the magnetic field strength is maximum, and freezes there in a thick gelatinous mass (Fig. 351).
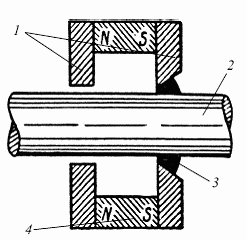
1 - tips; 2 - shaft; 3 – magnetic fluid; 4 - magnet
The shaft can also be non-magnetic, such as brass, titanium and even glass. Then the washers are brought closer to each other, and the gap between them is filled with magnetic fluid. Curled into a dense annular tourniquet, the liquid is pressed even against a non-magnetic shaft and seals it (Fig. 352).
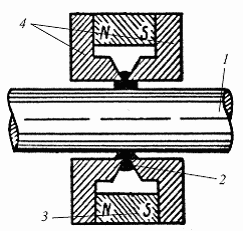
1 - shaft; 2 – magnetic fluid; 3 – magnet; 4 - tips
Magnetic fluid, especially oil, can be successfully used as a "perpetual" lubricant, filling it with both sliding and rolling bearings, even gearboxes and gearboxes, holding it in the right place with magnets (Fig. 353). In addition, such mechanisms are not only self-sealing with magnetic fluid, but also lubricated.

1 - magnet; 2 - bushing; 3 - shaft; 4 - magnetic fluid
The question arises: can the magnetic fluid in which the suspension is located magnetic particles, be a lubricant? Will she play the role of emery powder?
It turned out that no, and this has been proven by numerous experiments. The particle sizes are so small that they do not affect the surface finish of the rubbing parts in any way, as if they do not exist.
Magnetic fluid can play the role of not only lubrication, but also the bearing itself. If, during the rotation of the shaft, it is brought into rapid rotation with the help of special notches on the surface of the shaft, then even heavily loaded shafts will float in it (Fig. 354). Such bearings are called magnetohydrodynamic.

a - radial bearing; b - thrust bearing; F - forces
Magnetic fluid has another amazing, truly unique property. In it, as in any liquid, less dense bodies float and bodies more dense than itself sink. But if you apply a magnetic field to it, then the drowned bodies begin to float. Moreover, the stronger the field, the heavier bodies rise to the surface. By applying a magnetic field of different intensity, it is possible to make bodies float with some given density. This property of the magnetic fluid is now used to enrich ore. It is drowned in a magnetic fluid, and then, with a growing magnetic field, empty rock is forced to float, and then heavy pieces of ore.
There are even printing and drafting devices that run on magnetic fluid. A little magnetic fluid is added to the paint, and this paint is sprayed in a thin stream onto the paper stretched in front of it. If the jet is not deflected by anything, a line will be drawn. But electromagnets are placed in the way of the stream, like the deflecting electromagnets of a TV kinescope. The role of the flow of electrons here is played by a thin trickle of paint with a magnetic fluid - electromagnets reject it, and letters, graphics, and drawings remain on paper.
Magnetic fluid is also used to collect various petroleum products on the surface of the seas, oceans, lakes. It often happens that a person is not able to prevent oil pollution of the water surface, for example, in the event of an oil tanker accident, when a huge spot covers many square kilometers of the sea, polluting everything around. Purification of water from such contaminants is a very difficult, long and not always feasible task. But magnetic fluid helps here too.
A small amount of magnetic fluid is sprayed onto the spilled slick from a helicopter, which quickly dissolves in the oil slick, then strong magnets are immersed in the water, and the slick begins to shrink to a point, here it is pumped out by pumps. The water becomes clear again.
And what scope for magnetic fluids in medicine! Let us imagine that a certain part of the body is to be treated with some kind of medicine, without affecting the rest of the body. For example, it is necessary that it be concentrated in some organ of a person, and the blood carries it throughout the body. Having mixed the medicine on the magnetic fluid, it is injected into the blood, and then a magnet is installed near the sore spot. Naturally, the magnetic fluid, and with it the medicine, will soon gather near the magnet and will act only on the diseased part of the body.
Why not buy a magnetic jaw?
Talk about the healing properties of magnets, as well as methods of treatment with them, has been going on since ancient times.
However, these reports were contradictory. Here is what the "father of magnetism" Hilbert wrote about this. “Dioscorides teaches that a magnet with water mixed with honey ... is given to cure fatty moisture. Galen writes that a magnet has a power similar to that of hematite. Others say that the magnet causes mental breakdown, makes people melancholic and most often kills them. It is said by the East Indians that a magnet taken in small quantities preserves youth, so the old king Zeylam is said to have made bowls from the magnet in which to cook his food.
Gilbert himself, for example, believed that "in its pure form, a magnet can be not only harmless, but also have the ability to put in order too wet and rotting insides and improve their composition." It is not said too scientifically for Queen Elizabeth's life physician and one of the greatest scientists of her time, but there is some truth in this.
It is not for nothing that famous doctors of antiquity, such as the founder of medicine Hippocrates, the ancient Roman physician Galen, and the medieval physician Paracelsus, wrote about the magnet as a medicine. Magnets for the purpose of healing in ancient times were worn on the chest, on the belt, tied to the arms and legs. The ancient Egyptians crushed the magnet into powder and took it inside, believing that it kept youth. A contemporary and friend of the composers Mozart, Haydn and Gluck, the famous doctor from Vienna, Franz Mesmer, treated a wide variety of diseases with a magnet.
It all started with the fact that in 1774 Mesmer began to apply magnets to the sore spot of his patients, and unexpectedly for him, many diseases that had not been cured before began to disappear. In 1775, the Bavarian Academy elected Mesmer as a member for this.
Mesmer believed that the entire universe and living beings are saturated with a magnetic fluid or gas - fluid. A magnetic atmosphere spreads around a person, and magnetic poles are found on his body. If the fluid flows in the human body in the right direction, then everything is fine, but if not, then the person becomes ill. In this case, a magnet must be placed on the body in a certain place, which corrects the situation with its fluid, and the patient recovers.
The matter came to curiosities. For example, in 1780, a medical office was opened in London under the name "Castle of Health". The highlight of the healing program in this castle was the "star bed" located on forty large magnets. For huge money - 100 pounds - the patient could spend the night on the "star bed" and undergo the therapeutic effect of magnets.
In 1777, the French Royal Society of Medicine organized a commission that tested the success of the treatment with magnets, and came to the conclusion that "it is impossible not to recognize the therapeutic effect of the magnet." The magnet was especially recommended for the treatment of nervous diseases, convulsions, convulsions and headaches.
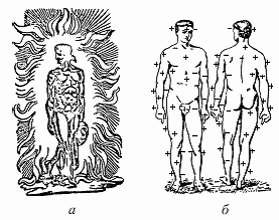
The French physician D'Urville magnetized water with a strong horseshoe magnet, and then treated his patients with this water. "Magnetic" water helped heal wounds, heal ulcers.
Dr. Durville believed that there is a kind of “magnetic atmosphere” around a person (Fig. 355, a), and the person himself has magnetism with its north and south poles (Fig. 355, b).
In the 19th century, homeopathic pharmacies in St. Petersburg and Moscow sold “healing magnets” to the fullest, for example, magnetic bibs (Fig. 356). Doctors, advertising these magnets, wrote:
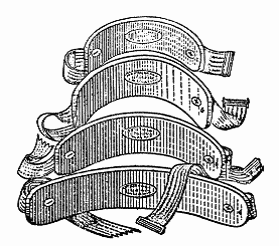
“With the help of magnets, life, which is dying out in a body exhausted from a long series of sufferings, is revived exactly from the influx of new forces.”
Recently, when they began to pay increased attention to the side effects of chemical drugs, doctors again attracted the old methods of treatment, including magnets - magnetotherapy.

Now magnetic bracelets (Fig. 357) are quite common, which serve to equalize blood pressure and have a calming effect on a person. Believe me, this is also a well-forgotten old one!
The author once sewed a strong rare earth magnet into the back of a tie and wore this tie around his neck for a long time. But blood pressure and well-being have not changed at all. But to a friend whom the author lent to wear a tie, he helped. Again contradictory evidence!
Of particular note are experiments to identify the effects of strong magnetic fields on humans and animals.
Back in 1892, a dog was placed between the poles of a strong magnet in Edison's laboratory, and when the researchers were convinced that nothing harmful had happened, they placed a boy there. (An experience that today would be considered a crime!) But nothing bad happened to the boy either. It was concluded that strong magnetic fields are harmless to the body.
One of these "reckless" experiments was described by the physicist V.P. Kartsev in his book on magnetism.
“I remember how a young engineer, having decided to prove the harmlessness of a magnetic field, stuck his head into the gap of an electromagnet of a powerful atomic machine.
- So how is it? they asked him.
- Nothing special. Only when you get out, like some kind of flash before your eyes, as if from a photographic "blitz".
Scientists call this flash phosphene. Most likely, it is due to the fact that when the magnetic field changes (when a person leaves the sphere of action of the magnetic field or enters it), “extraneous” biocurrents are induced in the brain tissues, distorting the usual picture.
However, careful studies of living organisms in strong magnetic fields have revealed changes in the blood and a number of other undesirable phenomena. Therefore, doctors recognized prolonged exposure to strong magnetic fields as harmful.
Speaking about the influence of magnets on people's health, one cannot fail to mention magnetic instruments and prostheses. Everyone knows a textbook example when a steel mote or shavings are removed from the eye with an electromagnet. They came up with this method more than 100 years ago in England. For the same number of years, the method of detecting and extracting metal fragments from wounds with an electromagnet has been known. In 1887, this method was tested on the American President J. Garfield, who was shot during the assassination attempt.
With magnetic probes, it is easy to remove accidentally swallowed steel objects from the stomach. At the end of this probe is a strong electromagnet fed externally through flexible insulated wires.
During the First World War, steel fragments were erupted from wounds with an electromagnet, and it was no longer fed with direct, but with alternating current, which facilitated the procedure.
At the same time, in 1915, a "magnetic arm" was invented in America, that is, a prosthetic arm with an electromagnet at the end. Powered by a battery, this electromagnet allowed a disabled person to hold various tools with iron handles.
Of interest is the magnetic prosthesis of the jaw, in which the teeth are made of strong magnets directed by the same poles to each other. Such a jaw is well kept in the mouth due to the repulsion of the magnet teeth. One thing is bad - it's worth gaping, as your mouth automatically opens!
Where did the North Pole go?
Sometimes, when they want to emphasize the inviolability of something, they compare it with a compass needle pointing north. Most people naively believe that this arrow really points to the north, and it always has been and always will be. Probably many of the readers of this book also think so.
It turns out that the compass needle does not point to the north at all, but to a place that, quite by chance today, turned out to be near the geographic North Pole - the South magnetic pole. The geographic poles are the exit points of the Earth's axis of rotation. The last time the South Magnetic Pole coincided with the North Geographic Pole quite accurately was in 1663. How scientists have established this, more will be said. And as for the magnetic poles of the Earth, in particular the South, he makes the most intricate, unpredictable travels around the globe today.
700 million years ago, this pole was located off the coast of modern California (point A, Fig. 358). Then it began to shift south, passed almost along the equator to the west, 200-300 million years ago ended up off the coast of Japan (again, modern!), only then turned north and in 1663 coincided with the geographic North Pole of the Earth (point AT).

This is according to European scientists. American paleontologists also dealt with the issue of the "travel" of the Earth's magnetic poles, but their results are very different from European ones. So, the Americans believe that this pole 700 million years ago began its journey from the middle of the Pacific Ocean (point A?), Then it shifted not so much to the south as to the west, passed through China, Mongolia and our country, described a loop near the Northern Geographic pole and came close enough to it (point B?). The current position of the South Magnetic Pole causes no disagreement among American and European paleontologists.
If Australian or African scientists had taken up the issue of the travel of the Earth's magnetic poles, then their data would also not coincide with each other.
What's the matter? It is clear that the Earth has only two magnetic poles and the South magnetic pole could move along only one single trajectory. What caused the discrepancies in the testimony of scientists from different countries?
Before answering this question, let's look at how scientists find the position of the Earth's magnetic poles in the distant past.
The study of the Earth's magnetic field in the past geological epochs, sometimes separated from us by hundreds of millions of years, or the Earth's paleomagnetism, is being studied by a young science - paleomagnetology, which means "the science of ancient magnetism." The research of paleomagnetologists is based on the study of the residual magnetization of rocks, which arose during their formation.
This happened during the eruption of molten rocks millions of years ago. Hot lava containing particles of iron, cooling in the field of terrestrial magnetism, received a magnetization depending on the position of the Earth's magnetic poles. This magnetization remained unchanged for millions of years and was measured by paleomagnetologists using modern precision instruments. Knowing the age of the rock and the direction of its magnetization, it is not difficult to establish the position of the Earth's magnetic poles during this period.
And it is possible to trace the movement of the magnetic poles over the past few millennia with very high accuracy - a huge amount of data on this is provided to scientists by ancient fired ceramics. Ceramic products, the age of which can already be determined with greater accuracy, retain their magnetism, obtained during cooling after firing. And if such a product did not fall into an accidental fire after its manufacture, then it served as irrefutable evidence of the location of the Earth's magnetic poles at its birth.
Sedimentary rocks, arising from the slow deposition of tiny particles in water, are also good witnesses to changes in terrestrial magnetism. These particles stay in the water for a very long time in a suspended state, like the arrows of a compass, they orient themselves in the Earth's magnetic field and settle in this position. This is how the magnetization of sedimentary rocks oriented towards the ancient poles of the Earth arises.
And most recently, the position of the magnetic poles is measured and recorded by precise instruments. So, for 20 years, from 1928 to 1948. The south magnetic pole of the Earth has shifted by 150 km! If it moved all the time at such a speed, namely 7.5 km per year, then in 100 million years it would travel along and across the entire surface of the Earth.
And now, when we already know about the variability of the location of the Earth's magnetic poles, let's return to the question of why the positions of these poles, calculated by scientists from different continents, do not coincide. Indeed, at one and the same moment of time, the position of the pole is one and only, and the error cannot be so large, unless, of course, the witnesses of paleomagnetism themselves are deliberately displaced. But by what forces can one artificially transfer hundreds and thousands of kilometers and expand enormous amounts of volcanic and sedimentary rocks at the same time? The only conclusion suggests itself: the continents themselves drifted along with these rocks, traveled huge distances, floating on the liquid and hot center of the Earth, like ice floes in the ocean. So, if you check the paths of this drift, mentally scroll the picture back hundreds of millions of years, then the continents will converge into one supercontinent called Gondwana, or Pangea (Fig. 359).
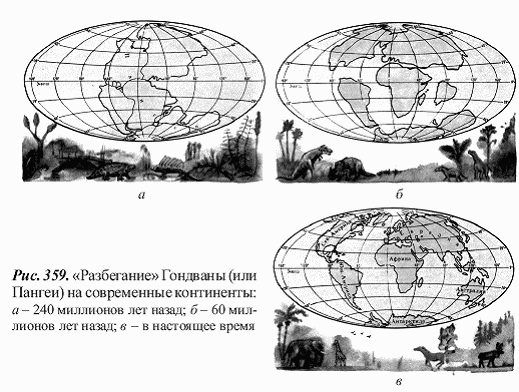
Who “stained” the Sun?
So, our Earth is still a magnet with two pronounced North and South poles, or a dipole magnet. Let these poles walk as they want, let them change places, let the Earth's magnetism weaken or strengthen, but the Earth is a magnet.
But the closest neighbor of the Earth - the Moon - is almost completely devoid of magnetism. This has been proven both by satellites and by instruments directly installed on the Moon. A magnetic compass would thus be useless on the Moon.
Mercury, the planet closest to the Sun, has a dipole magnetic field, similar to Earth's, but about 100 times weaker. Given that it is not very strong on Earth either, it is not easy to detect it on Mercury.
The planet Venus turned out to be almost completely devoid of a magnetic field, at least existing in the depths of the core. It is 10,000 times weaker than the earth. Of course, the powerful atmosphere of Venus reacts to solar plasma flows, and induced magnetism arises in it. But the planet itself rotates very slowly, and the absence of any kind of currents in its core, which generate a magnetic field, is associated with this.
Mars has a weak magnetic field, which can only be said to be slightly stronger than that of Venus and has a dipole character.
There are still five planets left. So far nothing definite can be said about the magnetic fields of Uranus, Neptune and Pluto, but Jupiter and Saturn have more than compensated for the paucity of information about the magnetic fields of the other planets.
On Jupiter - the giant planet - the most gigantic magnetic field. It, like the Earth, is bipolar - dipole, but almost 200 times stronger. It is interesting that on Jupiter, unlike the Earth, the magnetic poles are close to the geographical ones of the same name, that is, the South magnetic pole is in the Southern Hemisphere, and the North one is in the North.
The same picture is observed on Saturn. This planet itself is smaller than Jupiter, its liquid core is also small, so the magnetic field of Saturn is similar to the field of Jupiter, but weaker.
So, there is one more, the main body solar system- its center, the Sun itself. And although this star, the closest to us, is located very far away - almost 150 million km from the Earth, the magnetic field of the Sun has an extremely great influence on us. The most interesting phenomenon on the Sun in terms of its magnetism is the spots on its surface.
Sunspots were first noticed in 1611 by the Jesuit priest Scheiner, who, looking at the luminary through a telescope recently built by Galileo, saw dark spots on its surface. As was customary in a Jesuit order with its strictest discipline, Scheiner reported his observations to the general of the order. He did not even want to check the message of Shayner, advising him to keep quiet for his own sake. The time was such that it was possible to please the fire.
The attitude towards the Sun, as something ideal, was so strong that even the great Galileo did not believe Scheiner's message and declared: "The sun is the eye of the world and cannot suffer from thorns!"
It so happened that for almost 70 years, from 1645 to 1715. spots on the Sun practically did not appear, which greatly undermined the credibility of Scheiner's discovery. But you can’t hide an awl in a bag, they began to look at the Sun intently through telescopes and, finally, after a long break, they again saw spots there. There was nothing left to do but “forgive” them to the Sun, fixing this with the saying: “There are spots on the Sun!”
People began to wonder: how to explain the dark spots on the hot surface of the star?
In 1800, the famous English astronomer W. Herschel proposed a fantastic hypothesis, from which our contemporary may become uneasy. In the depths of the Sun there is intelligent life, it is cool there, and spots are dark areas of cold crust that appear through hot clouds. And this idea was considered a working hypothesis for almost a century!
Finally, in 1908, the American scientist D. Hale discovered that there is a strong magnetic field in sunspots - about 3,000 oersteds. On the rest of the surface, the field is thousands of times smaller, which gave reason to see in the spots the poles of the magnet that came out to the surface of the Sun.
It turns out that in the bowels of the Sun there are magnetic tube-rings parallel to the equator, formed by the same dynamo process as in the bowels of the Earth. Only on the Sun, due to various reasons related to its size and processes occurring in the depths, these tubes can have different directions. force field, and existing at the same time. Slowly rising to the surface of the Sun, these tubes open, forming the open ends of the poles of the magnet.
The total magnetic field of the Sun changes on an 11-year cycle, so that for 11 years the North magnetic pole is in the Northern Hemisphere, and the South - in the South. For the next 11 years, the polarity of the magnetic poles is opposite to the geographical one. But the most amazing thing is that this "polarity reversal" does not occur simultaneously, and for several months or a year the Sun turns into a huge monopole - a mysterious magnet with only one single pole.
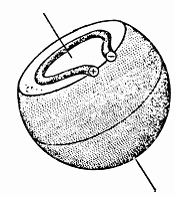
When the magnetic tube "emerges" from the bowels of the Sun, sunspots develop on its surface for several days (Fig. 360). First, at the point where the ends of the tube come out, one or several black dots visible through a telescope with a diameter of hundreds of kilometers appear, and then in one or two days they grow into a spot tens of thousands of kilometers in size.
Consequently, magnetism “stained” the Sun. And, looking ahead, let's say that these seemingly distant magnetic power spots on the star play a huge role in our life!
Earth echo of solar storms
The moment when spots appear on the Sun is characterized by a maximum of solar activity, which also has a period of 11 years. Solar activity, or solar storms, is associated with a number of earthly events that play a big role in our lives. This connection was most fully noticed, studied and described by the Russian scientist A. L. Chizhevsky. The scientist noticed that the most diverse processes on our planet usually have a cyclical nature and occur simultaneously, and these processes are closely related to changes in the magnetic activity of the Sun.
So, for example, the frequency of outbreaks of epidemics, diseases of animals and plants coincides with the period of solar activity. Chizhevsky publicly expressed his first thoughts on this matter as early as 1915 (Fig. 361).

“An astronomer reading the epidemiology of cholera is involuntarily amazed by the fact that the years of solar storms and hurricanes that are well known to him cause such great disasters, and, on the contrary, the years of solar calm and peace coincide with the years of man’s liberation from the boundless horror of this irresistible invisible enemy” .
To verify the authenticity of the connection between epidemics and the periodicity of solar activity, Chizhevsky used a method later called the epoch superimposition method. The scientist obtained an average solar activity curve for nine periods, and then inserted statistical data on cholera diseases in Russia in the same periods over the years. And a picture of a remarkable parallelism of two series of phenomena appeared before our eyes: solar activity and the development of cholera epidemics in Russia over 100 years (see Fig. 361, b). The same connection was traced for influenza epidemics (see Fig. 361, c) and a number of other diseases. It became possible to predict these epidemics and confront them fully armed.
What explains this mysterious connection between such seemingly dissimilar phenomena as solar magnetism and life on Earth? Chizhevsky discovered that the vital functions of pathogenic bacteria, as well as the resistance of humans, animals and plants to them, are directly related to electromagnetic disturbances in the atmosphere of the Sun and on Earth.
Subconsciously, the ancients also felt this, which is why they had the corresponding signs - signs. But the ancients could not explain the connection between natural phenomena and various disasters on Earth. They were fond of the poetry of comparisons, fell into mysticism. And the scientists of that time, criticizing all kinds of natural omens, did not take into account their real connection with mass diseases, natural disasters and other phenomena on Earth.
It's amazing how many aspects of our lives are not affected by sunspots! The frequency of thunderstorms and storms, the harvest of grain crops and other plants, even the birth rate of children are in a clear relationship with the activity of the Sun's magnetic field - sunspots.
Here is a short list of the main phenomena on Earth that are clearly associated with the appearance of sunspots:
1. The size of the crop of cereals (see Fig. 361, e) and the price of grain.
2. Harvest and quality of grapes.
3. Growth of wood (thickness of annual rings).
4. The time of flowering and splendor of flowering plants.
5. Mass diseases of humans, animals, plants.
6. Reproduction of animals, insects, fish.
7. The birth rate of children (see Fig. 361, a)
8. Time of spring arrival of birds.
9. The frequency of storms (see Fig. 361, d) fires and human being struck by lightning.
10. Frequency of accidents and crimes.
11. Frequency of disease exacerbations and sudden deaths.
All these phenomena are related to the number and area of sunspots, characterized by the Wolf numbers introduced by the Swiss astronomer R. Wolf. Moreover, the maximum of one phenomenon does not always generate the maximum of another. Thus, wheat prices become maximum during the years of minimum Wolf numbers - sunspots.
Speaking about human diseases and their connection with sunspots, Chizhevsky warns that it would be completely wrong to believe that diseases are caused by these spots. It's about only about a push from the outside, the influence of the magnetic activity of the Sun, the flow of particles and electromagnetic radiation reaching the Earth during this period, on the human body. And if for a healthy, young person this influence is negligible, then for a person weakened by a long illness or infection, it can be decisive.
Chizhevsky not only revealed this influence on individual parts and departments of the human body, but also taught how to protect oneself from this influence. “Science can speak loudly enough here. Yes, physics knows ways to protect a person from such harmful influences of the Sun or similar ones, wherever they come from. Metal is the savior here: iron, steel, lead… It is easy to calculate the thickness of that metal screen that will protect sick and old organisms.”
It turns out that thin sheets of metal, a fraction of a millimeter thick, are enough. Chizhevsky proposes to sheathe the walls, floor and ceiling of the hospital wards, where seriously ill patients lie, with such metal sheets that protect against the effects of electromagnetic radiation from the sun. On a signal from astronomical observatory about the approaching magnetic storm on the Sun, patients should be placed in such protected wards, where they will be one, two or three days, until the crisis of the disease has passed or the activity has fallen magnetic phenomena in the sun.
And, finally, about the absolutely fantastic influence of solar activity on our Russian events of the recent past. According to the calculations of the English astronomer D. Whitehouse, which were published in the 80s of the XX century, maximum number sunspots should have come in August 1991. What we had in Russia at that time, probably everyone remembers: on August 19-21 there was a coup by the State Emergency Committee. Thus, Whitehouse's calculation turned out to be amazingly accurate ...
In search of a magnetic monopole
Somehow, in childhood, the author came up with an unusual idea: to get a magnet with one pole - a monopole. The author had a large horseshoe-shaped magnet, and one of its edges, like half of the compass needle, as the author believed, tends to the south, and the other to the north. The middle of the magnet does not attract iron, and therefore does not tend to go anywhere. And it seemed that if you break the magnet and put the pieces on the wheels, or even better on the boards in the water, then one part of the magnet will float to the south, and the other to the north! Having made huge halves of the magnet and putting them on a truck or boat, it will be possible to get even to the North Pole, even to the South without an engine!
Of course, the magnet was broken and the red half was placed on a piece of board floating in a basin of water.
But the piece of magnet never floated anywhere. It only slowly turned around, so that its whole edge began to point to the south, and the broken one - to the north! Bringing the compass closer to the broken edge, the author, to his surprise, was convinced that on it ... the South Pole. And on the other piece, the broken edge became the North Pole. The nail began to be attracted equally strongly to each edge of the pieces, as if it was not this same nail that refused to be attracted when the pieces were together. Here are miracles!
Where did the poles go? After all, the nail was strongly attracted to each of them. And instead of pulling twice as hard, he didn't want to do it at all. The author felt completely fooled: both the magnet is broken, and the trip to the North is canceled, and there is no way to separate the North Pole of the magnet from the South ...
A magnet with a single pole, or, as it is called, a monopole, it turns out, does not contradict science at all. And it is surprising that we still do not have it, just as we do not have, however, evidence of its impossibility. Let's talk about this amazing magnet in more detail.
In 1931, the outstanding physicist P. Dirac (1902-1984) mathematically proved the possibility of the existence of a particle with a magnetic charge - a monopole, that is, that there can be separately "northern" and "southern" magnetic charges. Similarly, Dirac predicted the existence of the electron with positive charge- the positron, and already in 1932 it was discovered in nature.
But so much time has passed, and the monopole is still a ghost that exists only on paper. The fact is that the existence of a monopole gives a natural explanation for some physical phenomena that cannot be explained otherwise than with the help of a monopole.
From time to time, physicists set off in search of a monopole, but so far each time unsuccessfully. Magnetic and electrical phenomena are similar in almost every way, except for one thing. Electric charges - positive and negative - are enough to create both electricity and magnetism (the latter occurs when charges move). But electricity has a source of its existence - an electric charge, but magnetism has no charge. There is asymmetry, electricity has some advantages over magnetism.
And just Dirac proved that electricity does not and cannot have such an advantage. Magnetic and electrical phenomena must be completely similar, symmetrical. In this case, a fairly accurate portrait of the monopole was obtained. The unit charge of the monopole is 38.5 times greater than the unit charge of the electron. The interaction of two monopoles is 4,700 times stronger than the interaction of two electrons; therefore, at the same speeds as an electron, a magnetic monopole ionizes atoms 4,700 times stronger environment. Such a large magnetic charge makes it easy to control the monopole even in weak magnetic fields, to accelerate it to gigantic energies inaccessible to electrons. The monopole can work miracles in electronics, physics, and finally, be used, for example, in television, accelerator technology, and who knows where else.
They searched for monopoles in a beam of accelerated particles during their collision with matter, in cosmic rays. It was not possible to pull them out with the help of powerful magnets from iron-bearing rocks and meteorites, where monopoles of cosmic origin could get stuck and accumulate over millions of years. The monopole must be very stable, it will not disappear until it collides with another monopole of opposite sign. Even in the lunar soil they searched for a magnetic monopole, but to no avail.
Here and there sensational reports appear about the "capture" of a mysterious monopole, but upon careful verification, they turn out to be untenable. About 30 years ago, Chinese scientists were sure that they had discovered a monopole, but, alas, the discovery did not take place. In 1982, at the reputable Stanford University (USA), it would seem that they had already “caught” a monopole with the help of a superconducting magnet. But repeated experiments, more accurate, yielded nothing.
Finally, in 1985, at the University of London, with the help of the most sensitive sensors, it would seem that they discovered a monopole. But confirmation of this discovery has not yet followed.
What is the reason for such a long failure of the search for a monopole? Maybe it's very rare? Or are they not looking for it? Or did Dirac make a mistake and incorrectly determine the charge of the monopole? In this case, it turns out that they are not looking for something?
But there is no proof of the impossibility of the monopole either, and confirming this impossibility is no easier than finding the monopole itself.
Hurry, reader, seek and find your monopole. It would be a shame if someone else did it!
Amber with a magnet - brothers?
It turned out that this is close to the truth, and their lightning “brothered”. Indeed, when amber is electrified, sparks arise, and sparks are small lightning.
But lightning is lightning, and what does the magnet have to do with it? It was the lightning that turned out to be what united the amber and the magnet, previously "separated" by Gilbert. Here are three excerpts from a description of a lightning strike that show a close connection between the electricity of amber and the attraction of a magnet.
“... In July 1681, the Quick ship was struck by lightning. When night came, it turned out, according to the position of the stars, that of the three compasses ... two, instead of pointing north, as before, pointed south, the former northern end of the third compass was directed to the west.
“... In June 1731, a merchant from Wexfield placed in the corner of his room a large box filled with knives, forks and other items made of iron and steel ... Lightning entered the house precisely through this corner in which the box stood, broke it and scattered all the things that were in it. All those forks and knives… turned out to be highly magnetized…”
“... A strong thunderstorm passed in the village of Medvedkovo; the peasants saw how lightning struck a knife, after a thunderstorm the knife began to attract iron nails ... "
Lightning strikes, magnetizing axes, pitchforks, knives, other steel objects, demagnetizing or remagnetizing compass needles, were observed so often that scientists began to look for a connection between electric sparks and magnetism. But neither the passage of current through the iron rods, nor the impact on them of sparks from Leyden jars gave tangible results - the iron was not magnetized, although accurate modern instruments would probably feel this.
The compass needle deviated slightly in the experiments of the physicist Romagnosi from the city of Trent, when he brought the compass closer to the voltaic column - an electric battery. And then only when a current was flowing through the voltaic column. But Romagnosi then did not understand the reasons for this behavior of the compass needle.
The honor of discovering the connection between electricity and magnetism fell to the Danish physicist Hans Christian Oersted (1777-1851), and even then by accident. It happened on February 15, 1820, that's how. Oersted was giving a lecture on physics that day to students at the University of Copenhagen. The lecture was dedicated thermal action current, in other words, heating the conductors through which flows electricity. Now this phenomenon is used all the time - in electric stoves, irons, boilers, even in electric lamps, the spiral of which is white-hot by current. And in the time of Oersted, such heating of a conductor by current was considered a new and interesting phenomenon.
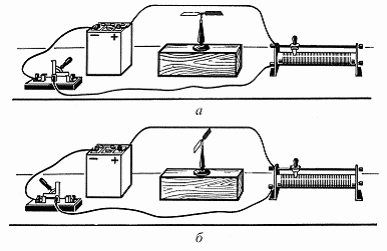
a - the battery is disconnected, the compass needle is parallel to the conductor; b - the battery is on, the arrow turns perpendicular to the conductor
So, the first accident consisted in the fact that near one of the heated conductors there was a compass, which was completely unnecessary in such experiments. Then another accident occurred - one of the students surrounding Oersted noticed that the compass needle deviates when the conductor is connected to a current source - an electric battery (Fig. 362). And the third accident completed the birth of the discovery - the student decided to point out to the eminent professor a seemingly completely extraneous phenomenon that had nothing to do with the topic of the lecture, and the scientist listened to the student's remark.
It is a pity that we do not know the name of that student - after all, he is a full-fledged co-author of the great discovery - the connection between electricity and magnetism, the official author of which was Professor Oersted.
Oersted conducted a whole series of experiments explaining the laws of behavior of a magnetic needle. It was noticed that the flow of current through the conductor in different directions causes a change in the direction of deviation of the compass needle. Oersted also noticed that no isolation interfered with this behavior of the arrow. The scientist placed a variety of insulating materials between the conductor and the compass, but the arrow deviated in the same way, and its deviation depended only on the direction and strength of the current passing through the conductor.
And then, with great haste, Oersted published his famous "pamphlet" - four pages of text on Latin understandable to most scientists. This pamphlet made a staggering impression on academia. Oersted's experiments began to be repeated in many laboratories, and there was no limit to the surprise and delight of those present. Witnesses recall that one of those present at such an experiment stood up and excitedly said: “Gentlemen, there is a revolution ...”
Awards and honors rained down on Oersted. He is elected an academician of the French Academy and is awarded a prize established at one time by Napoleon Bonaparte for major discoveries in the field of electricity - 3 thousand gold francs, he is appointed a member of the Royal Society of London and many other scientific societies. In Oersted's homeland, King Frederick VI awarded him the Grand Cross of the Dannebrog Order, Denmark's highest award, and allowed him to establish the Polytechnic Institute. Oersted opens a society in Denmark to "encourage scientific pursuits" and even begins to publish a literary magazine. By the way, the love of the venerable scientist for literature was not in vain for literature itself: Oersted patronized "little Hans Christian" - the future great storyteller Andersen.
Oersted becomes a national hero in Denmark and the most popular scientist in Europe. Hans Christian Oersted died in 1851 at the height of his fame.
How did the electromagnet gain strength?
One discovery breeds another. As soon as Oersted noticed the connection between electricity and magnetism, in September of the same 1820, the French physicist D. Arago noticed that the wire through which current flows attracts iron filings and magnetizes steel needles just like a magnet. A.M. Ampère, a scientist whom we all know at least by the unit of current named after him, once entered Arago’s laboratory. Ampere proposed to roll the wire into a spiral, and place the needle inside the spiral. The scientists immediately carried out the experiment and were more than rewarded for it - the needle was magnetized much stronger than before. The resulting spiral, or tube, later called a solenoid, is now well known to any schoolchild. The word "solenoid" is translated from Greek and means - "tubular", "in the form of a tube". But the genius of Ampere did not stop only at the creation of the device. Having caught the connection between the magnet and the solenoid, Ampere suggested that inside the magnet there are a huge number of tiny current-carrying rings. It is now known for certain that Ampère's idea was correct - the role of rings with current is played by electrons rotating around the nuclei of atoms (Fig. 363).

A new era began in the understanding of many physical phenomena and processes, where current and magnet played the main roles. And, perhaps, these two concepts nowhere show their connection so closely as in an electromagnet. The Ampere solenoid was not yet an electromagnet in the direct sense of the word - there was no iron core, which became a real magnet when current was passed through the turns of the wire. This core greatly enhanced the action of the solenoid, making the electromagnet much stronger than the best natural magnets.
And in order to simply insert the core into the solenoid, it took 5 years, and the discovery was made by the English mechanic William Sturgeon (1783-1850) in 1825.
Sturgeon's biography once again shows that a creative person will not disappear, no matter the conditions in which his childhood and youth pass. Such examples are widely known to us among writers, artists, musicians, and among inventors and scientists.
No one was engaged in the upbringing of little William. His father, a rather frivolous person, did nothing but fish and amused himself with cockfights. Starting to learn shoemaking, William soon ran away from his harsh teacher, who starved him. The young man worked in the police, and then served in the army. But during the service, he managed to make simple experiments in physics and chemistry.
There, in the army, an event took place that had a great influence on the young soldier. Sturgeon witnessed an unusually strong thunderstorm. Huge blinding lightning and thundering thunder struck him, and he decided to study electricity.
But in order to read books, you need to be literate, and Sturgeon began to study hard reading, writing and grammar, gradually mastered mathematics, physics, languages, and besides, he drew and repaired watches with pleasure. And all this in the army with its discipline, mainly at night!
After completing his military service, the young Sturgeon bought a lathe and began to manufacture physical and electrical appliances. This happened in 1820, when the great discoveries of Oersted, Arago and Ampère were made. And on May 23, 1825, Sturgeon presented the first electromagnet he had built to the Society of Arts.
It was a horseshoe-shaped rod, varnished for electrical insulation, 30 cm long and 1.3 cm in diameter. Only one layer of bare copper wire was wound on this rod, which was connected to an electric battery (Fig. 364). With a mass of 0.2 kg, Sturgeon's electromagnet lifted an iron load, almost 20 times heavier. The first electromagnet immediately turned out to be stronger than natural magnets of the same mass.
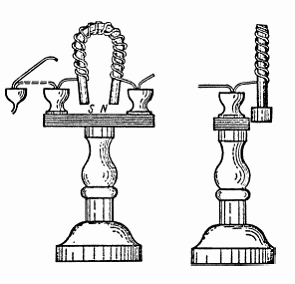
The Board of the Society of Arts was able to evaluate Sturgeon's work. He was awarded a medal and a cash prize, and the device was exhibited in the museum. However, despite Sturgeon's subsequent outstanding achievements, fame and success never came to him. He died in poverty and deprivation in 1850, and not even a portrait of the inventor of the first electromagnet has been preserved.
For a long time, until 1840, Sturgeon's electromagnets were the strongest in the world. And then Sturgeon's student, the future great physicist D. Joule, stepped forward. By increasing the number of electromagnet poles and rationally placing them on the load, he creates a structure capable of lifting 1.2 tons with its own weight of 5.5 kg! It is important at the same time that the poles are paired and their number is even.
It should be said that not any increase in the number of poles is beneficial. So, for example, a "three-legged" magnet (Fig. 365, a) is worse than the usual two-pole one (Fig. 365, b), because the magnetism of each of the rods interferes with the others. It is also unprofitable to make up one large magnet from separately wound small ones.
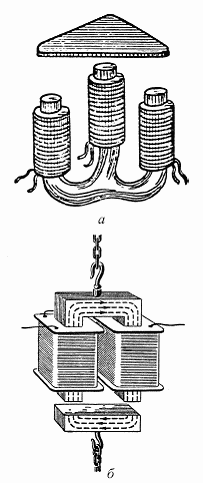
Electromagnets have become widely used in industry for lifting heavy steel loads (Fig. 366). In 1864, an electromagnet weighing 260 kg was built in New York, "which lifted seven people once, and no one knows how much more it can lift."
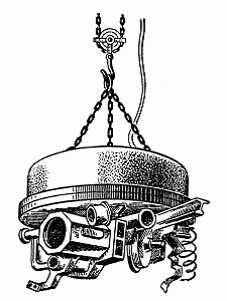
Note that the electromagnet was not a very safe lifting device. As soon as the current stopped, the electromagnet instantly lost its power, and a terrible load fell "from the sky" onto anything and anyone. And there could be plenty of reasons for the interruption of the current - the wire broke, the fuse was knocked out, there was an accident at the station, etc. Therefore, in the future they began to act differently.
The coils of wire began to be wound not on simple iron, but on a magnetized material - a permanent magnet, and in such a way that when a current was passed, it would be demagnetized. To lift the load, the current was turned off, and a permanent magnet (and now there are very strong permanent magnets) attracted steel, iron and cast iron objects, which were lifted and carried into place. And in order to release the load, current was applied to the coils, and the magnet was temporarily demagnetized - the poles of the permanent magnet and the solenoid windings were opposite! The cargo was unhooked. When the magnet did not need to work, the current, of course, was turned off by moving the magnet away from iron objects, for example, by lifting it into the air.
Cranes with such a magnet have become much safer, they are no longer afraid of interruptions in the supply of current.
In the first half of the XX century. electromagnets were built that lifted loads up to 75 tons. It seemed that the strength of electromagnets could grow indefinitely ... However, it turned out that the benefit of introducing an iron rod into the winding by Sturgeon began to gradually disappear. While the coils were small (remember the single-layer winding of the first electromagnet), iron greatly increased the lifting force of the magnet. But then the creators of electromagnets noticed that with an increase in the strength of the magnet, its iron seems to be saturated and no longer helps the electromagnet. They began to build magnets with short pointed poles, a massive yoke and huge coils, since these measures, as it turned out, increased the lifting force even more.
It is possible, of course, to make the amount of iron in the electromagnet so large that it is not brought to "super-saturation". The American inventor Edison, for example, proposed to build the largest electromagnet in the world by wrapping wire around a rock of magnetic iron ore in the American city of Ogden, weighing more than 100 million tons!
Unfortunately, this bold and ingenious project was not carried out, otherwise the legend of a magnetic mountain pulling nails out of ships would have come true!
Electromagnetic tricks and scams
Let's talk about "frivolous" applications of electromagnets.
First of all, these are circus tricks. Also in late XIX in. a certain trainer showed a "scientific" elephant, who supposedly could do complex mathematical calculations in his mind and give the correct answers. The trainer loudly asked the elephant questions related to any mathematical operation. After that, he took a pointer with his trunk and really pointed it at some number on the board in front of him. This figure always turned out to be correct, which should have indicated the high mathematical training of the elephant and the fact that he understands the question posed in human language.
The solution to this trick is simple. An electromagnet was attached below each number on the board. The mathematical actions assigned to the elephant were performed by the trainer himself or his assistant, who closed the winding of the electromagnet lying under the corresponding number. The elephant only had to take an iron pointer in his trunk and drive it near the board with numbers. When the pointer approached the switched on electromagnet, it itself, without the slightest participation of the elephant, was attracted to the correct figure. Now any schoolboy would have guessed about the deception, but more than a hundred years ago, electromagnets and their properties were not so widely known, which caused the sensational success of the mathematician elephant.
Moreover, electromagnetic phenomena were completely unknown to the peoples of Africa at that time. This allowed the Europeans to easily mystify them with simple tricks. One of these tricks, "proving" the advantage of white people over the local population, was shown by the French magician Robert Goodin. This not harmless trick, which helped the French enough in the conquest of Algeria, is colorfully told by Robert Goodin himself.
“On the stage is a small wrought iron box with a handle on the lid.
I call a stronger person from the audience. In response to my challenge, an Arab of medium height, but strong build, comes out, representing an Arabian Hercules. He comes out with a cheerful and presumptuous look and, smiling a little mockingly, stops beside me.
“Come here,” I said, “and pick up the box.
The Arab bent down, picked up the box and arrogantly asked:
- Nothing else?
- You are now weaker women. Try lifting the box again, I replied.
The strong man, somewhat unafraid of my charms, again took up the box, but this time the box resisted and, despite the desperate efforts of the Arab, remained motionless, as if chained to the place. The Arab tries to lift the box with enough force to lift a huge weight, but all in vain.
A strong electromagnet was installed under the carpet on which the box stood, and the box itself, or at least its bottom, was iron. The Frenchman easily lifted the box because the electromagnet turned off at that time. Knowing what was going on, the Arab could easily put the Frenchman to shame: by picking up the box for the first time, put it in another place, away from the magnet. But ignorance let the strong man down.
And here is the case when a foreign military department turned out to be deceived, and a certain charlatan inventor deceived him. He, unlike other charlatans, did not hide his invention and offered to check it in work at any time.
The experience that the inventor demonstrated was as follows. A heavy iron ingot was placed on a small pinch of the super-strong explosive allegedly invented by him. The pinch was undermined by an electric discharge, for which the author turned on the switch, and the explosion threw the heavy ingot to the ceiling.
The experience created a sensation - still, no one had ever seen explosives of such power. The inventor was given a large sum of money to continue the experiments, and he fled.
And the secret was explained simply - a very strong electromagnet was secretly installed over the ingot in the laboratory where the experiment was carried out. Turning on the switch, the cunning inventor let current into the magnet winding - and the load flew up, supposedly from the force of the explosion. If the switch was turned off for a moment, the ingot would “stick” to the magnet, and everyone would understand what was happening. But the inventor, apparently, was dexterous at hand ...
The author himself in his youth had to play a similar joke with his acquaintances, of course, more harmless. The author's childhood and youth passed in Tbilisi, where the oriental backgammon game was probably even more popular than our traditional "goat". There was no yard where in the evenings, and even during the day, surrounded by a crowd of fans, the players would not play backgammon. The game is simple, but all the player's happiness depends on the number of rolled dice. Two sixes, or du shash, as they are called in the East, are especially valued. An Eastern proverb even says: “What should a good backgammon player do if the doo shash does not fall out in time?”
The author was not a good player, moreover, he was, apparently, a bad player, because he decided to win not at the cost of countless training, but ... with knowledge of physics. Although such an application of knowledge can be called a rather harsh word, but the desire to win did its job.
The author drilled holes in the dice under the black-painted recesses exactly opposite the six, inserted pieces of a nail there and again filled the dots with paint. Nobody could see the trick. And in his backgammon, the author inserted a small electromagnet powered by a battery under the middle of the board. The author agreed to play only on his "happy" backgammon. Thus, the author could throw out record doo shash at any time, guaranteeing himself a victory. The whole trick consisted in imperceptibly pressing a hidden button in backgammon - the bones tightly became in the position of souls. Needless to say - the author earned a huge fame. The best backgammon players of the quarter came to fight and left shamed. The insidiousness of "magnetic" backgammon was not only that the author could organize a shower for himself at any moment. In backgammon there are also such moments when it is impossible to think of a worse score - the chips are not put in the appropriate position and the move is generally lost, the player becomes defenseless. It is only necessary to imagine the desperation of the player - the opponent, to whom the author "slipped" a scarce soul at a time when he did not need it at all.
How did the cold help the magnet?
At the end of XIX - beginning of XX centuries. scientists managed to convert all gases without exception into liquid, and even the champion among them - helium. Its boiling point is only 4.2°C above absolute zero, which is minus 273.16°C. Now it is customary for scientists and engineers to measure temperature not in degrees Celsius, but in degrees Kelvin, which are counted from absolute zero, with 0 K = - 273.16 ° C. The boiling point of liquid helium, therefore, will be 4.2 K (the symbol "°" when measured in degrees Kelvin, unlike degrees Celsius, is not written).
The honor of obtaining liquid helium belongs to the Dutch scientist Geike Kammerling-Onnes, and a phenomenon directly related to magnets, the phenomenon of superconductivity, is associated with his name. Superconductivity should make a real revolution in technology, a significant role in which will belong to superconducting magnets.
At the beginning of the 20th century, or rather, until 1911 and the discovery of superconductivity, scientists did not know at all how current conductors, primarily metals, would behave when they were cooled.
Some scholars believed that electrical resistance conductors with decreasing temperature will constantly fall, and at a temperature of absolute zero it could disappear altogether. (This phenomenon is called superconductivity). But since absolute zero is practically unattainable, then superconductivity, therefore, cannot really be obtained. Others insisted that even at absolute zero, some resistance would remain due to defects in the metal crystals. And still others even argued that when approaching absolute zero, the resistance of conductors increases. And all this was proved theoretically.
And Kammerling-Onnes, with his famous experiment, showed that both of them, and others, are wrong, and the result is what it was difficult to expect.
In the spring of 1911, the scientist decided to freeze mercury in the liquid helium he had recently obtained. This helium was stored in a vessel, which was invented by the Englishman Dewar and which was later named after him.
Both cold and hot objects, including liquids, are equally well stored in Dewar vessels, since they are well protected by vacuum from heat entering from the outside and exiting from the inside. And the mirror layer makes it impossible to transfer heat by rays.
So, a tube with mercury was placed in a Dewar vessel containing liquid helium, which immediately froze there, and then Kammerling-Onnes passed a current through the mercury and measured the electrical resistance, just as radio engineers and electricians do today. The resistance of the mercury column decreased with decreasing temperature, until this temperature dropped to 4.12 K. At this temperature, the resistance abruptly disappeared altogether! Yes, no one even suspected this!
A conscientious scientist repeatedly changed the conditions of the experiment: either he took contaminated mercury, or he thinned and lengthened the column of mercury so that the resistance became more noticeable, but the result was still the same: the resistance is zero!
And finally, two years later, Kammerling-Onnes makes a decisive experiment, for which accuracy is no longer important measuring instruments. He makes a winding from lead wire and gives a current pulse. Lead also turned out to be a superconductor, and already at 7.2 K. If there is at least some, even negligible, resistance, then the current in the winding will die out very quickly, in a split second. The current in the winding did not fade at all!
So, superconductivity is open! And not at an inaccessible absolute zero, but at real temperatures.
For those who do not believe that the resistance of a superconductor is really zero, we can talk about an interesting and instructive experiment set by American physicist S. Collins.
He made a superconducting ring, like Kammerling-On-nes, placed it in liquid helium and ran a current through it. In a silver ring, for example, this current would die out in a few tenths of a second, and silver is the best known conductor. And in the Collins ring, the extinction of the current for 10 years could not be established. It takes at least 100 thousand years to notice this extinction with the most accurate instruments!
Meticulous physicists have calculated that before the current is completely attenuated, when it can no longer be measured by instruments, time will pass, billions of billions of times longer than the time of existence of our Universe! Is this not a total lack of resistance? And even then they say that scientists made such a conclusion unreasonably - some, negligible attenuation of the current is observed due to the stretching of the ring through which this current flows. It is known that the current flowing through the ring creates magnetic forces that tend to break the ring.
So this stretching and the associated drop in the magnetic field strength were mistakenly taken for the attenuation of the current. In fact, the current in the superconducting ring will flow forever, and we will get an eternal electromagnet!
Scandal and sensation in the physics of superconductivity
Scientific authorities, it turns out, can move science not only forward and backward, but even stop it in place for decades. This is exactly what happened in the 1930s. 20th century with the study and practical use of such an important phenomenon as superconductivity.
In 1911, the Dutch scientist G. Kammerling-Onnes unexpectedly discovered the phenomenon of superconductivity, when, upon strong cooling, almost to absolute zero, the electrical resistance of some metals drops to zero.
But nature, which so pampered the scientist with an unexpected discovery, prepared an unpleasant surprise for him here. As long as the current in the superconducting coil was small, everything was fine. But as soon as the current increased, it destroyed superconductivity itself. And that's not it. The magnetic field generated by the current in the winding, even a small one, 1,000 - 1,500 oersteds, also killed superconductivity. And then, perhaps, the most unfortunate story happened, literally a scandal in the study and application of superconductors. The well-known and respected physicist of that time, W. Keesom, theoretically proved that in the presence of a magnetic field, even the smallest currents will “switch off” superconductivity. It was Keezom's mistake.
The authority of the famous physicist played its role, and everyone believed that there was no question of more or less suitable superconducting magnets. Work in this direction was stopped, and scientists took up other, more practical, from their point of view, problems. But in vain! Dozens of years have been lost, and the loss in money is difficult even to estimate. But in the future, nature gave us a pleasant surprise.
A real sensation occurred in 1986, when the Swiss physicists D. Bednorz and K. Müller announced that they had created superconductors at temperatures above the boiling point of liquid nitrogen (77.4 K!). The message was so shocking that scientific journals initially refused to publish it.
Liquid nitrogen is extremely cheap, as they say, even cheaper than lemonade, it is a by-product in the production of oxygen, and it is simply often poured out and thrown away. Obtaining superconductivity at "nitrogen" temperatures was a dream of researchers and engineers, it seemed unrealizable. Hence the boom that rose after this message. Now scientists have already moved from enthusiasm to action, systematic research has begun in the field of high-temperature superconductivity, including in our country. As a result, materials were obtained that acquired the property of superconductivity at 100-110 K. There were reports of materials losing electrical resistance at almost ordinary temperatures of our middle band - from -20 to +10 °C. But, as it turned out, it was not superconductivity, but simply a strong, by hundreds and thousands of times, a decrease in resistance, which, although good, is fundamentally different from superconductivity.
What are these materials that have such tempting properties?
Unlike low-temperature superconductors, these are not metals, but ceramics, most often based on the elements yttrium and barium. The very procedure for making superconducting ceramics is extraordinarily simple and, as one well-known physicist put it, "surprisingly foolproof."
The components themselves that make up the new superconductors, although they are called rare earth, are by no means rare. They are part of the polymetallic ores, but due to the lack of demand, they have not yet been extracted from there, but went to the dump. So now we need to organize the processing of dumps of these ores.
Where can new superconductors be used? Power applications of superconductors will have to wait for now. But the unique properties of superconductivity, not associated with high currents, can already be used. For example, in microelectronics and computer technology, new superconductors can be used right now, since large currents are not required there.
There were earlier attempts to use superconductors for the needs of microelectronics and computer technology, even some elements were developed (a superconducting key, a superconducting memory cell - a cryotron), but their wide distribution was hindered high price cooling down to operating temperature. The need to cool to nitrogen temperature is not a problem. Moreover, it is even useful, since at the same time the noise level is reduced.
Nature, with her gifts, has not yet fully redeemed Keesom's arrogant mistake and our admiration for the authorities of science. We can confidently expect the imminent appearance of already "power" superconductors operating at temperatures that are usual for us. What can we get from this, while it is even hard to imagine!
How did Faraday outsmart Ampère?
Here we again need to return to the 19th century, to the famous experiments of Faraday (1791-1867). Immediately after Oersted's experiments, where electricity gave rise to magnetism, Faraday wrote in his diary the motto: "Turn magnetism into electricity." For 11 years, Faraday did not succeed. For many years in a row, the scientist constantly carried with him a spiral of copper wire and an iron core, doing the most incredible manipulations with these objects. But nothing good came of it, and in his laboratory journal "On the excitation of electricity by means of magnetism" the entry again appeared: "No result." Faraday devoted a special paragraph to each experiment, and the last paragraph in the journal is marked with the number 16041!
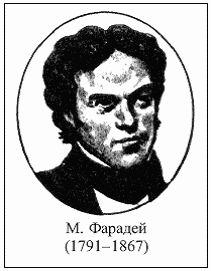
Faraday's fabulous performance and obsession was finally rewarded, and on August 29, 1831, he "attacked the trail." The whole of September and October were a continuous repetition in different versions of the same experiment, which laid the foundation for all electrical engineering. Here is how Faraday himself described this experience in his journal:
“I took a cylindrical magnetic bar and inserted one end of it into the lumen of a spiral of copper wire connected to a galvanometer. Then, with a quick movement, I pushed the magnet inside the spiral to its entire length, and the galvanometer needle experienced a push (Fig. 367). Then I also quickly pulled the magnet out of the spiral, and the arrow swung again, but in the opposite direction. These swings of the arrow were repeated every time the magnet was pushed or pushed out ... ”The scientist’s brilliant conclusion followed:“ This means that an electric wave arises only when the magnet moves, and not due to the properties inherent in it at rest.

1 - galvanometer; 2 - magnetic bar; 3 - a spiral of copper wire
Now we are well aware that if you put a magnet near the winding, or even push it into a spiral and leave it there, then expecting a current to appear with a stationary magnet is tantamount to believing in the appearance of energy from nothing. Indeed, a magnet lies inside the winding, does not lose anything, and current flows there, doing work at least to heat this winding. So it’s not far from the “perpetual motion machine”! True, as we have already seen, such a case is possible when the winding is superconducting - there the current that arose during the introduction of the magnet will flow forever - there are no losses! But the same effect was expected in those days, and not by anyone, but by Ampere himself and, perhaps, at first, by Faraday.
Simultaneously with Faraday, experiments on pushing magnetic cores into a wire spiral were also carried out by Ampère. To avoid the influence of the magnet on the sensitive galvanometer, both Faraday and Ampère placed the device in another room. At the same time, Ampere first placed the core inside the spiral and then went to the next room to check if the current had appeared. But, alas, the spiral was not made of a superconductor, but of an ordinary copper wire, and the current almost instantly faded out as soon as the core stopped moving. And Faraday entrusted the supervision of the device to an assistant, who noticed the appearance of current during the movement of the magnet. It would seem that it was worth Ampère to use someone's help or, at worst, to put a galvanometer in another corner of the same room and watch it himself!
Such unfortunate cases are quite frequent in the history of science, which gave rise to the great German physicist Hermann Helmholtz to exclaim: “And a great discovery depended on these accidental circumstances!”
This saying of Helmholtz fully applies to Faraday himself. Even 9 years before the opening of electromagnetic induction(namely, so they began to call the excitation of electricity by a magnet) Faraday was unusually close to him.
Observing the wire with current, doing intricate manipulations with it, Faraday unexpectedly discovered that the magnet begins to move near the wire with current. A handwritten drawing by Faraday illustrating this experiment has been preserved (Fig. 368). A magnet floats in a bowl filled with mercury. Mercury is connected to one pole of the current source, and in the same mercury is the end of the wire connected to the other pole. When an electrical target was shorted through the mercury, the magnet or the end of the wire would spin. This was the first unipolar electric machine, the principle of which the author himself did not understand at that time. And this is not the point - scientists were able to explain the operation of such a machine only much later.

But one way or another, it was Faraday who connected the magnet and the movement, having received both the first electric motor - the magnet rotates when current is passed - and the first electric generator - the winding gives current when the magnet moves around it. The beginning of the era of electrical engineering, without which modern technology is unthinkable, was laid!
What rotates a self-rotator?
After the remarkable discoveries of Faraday, there was only one step left to create electric machines.
What is an electric machine? These are motors that convert electric current into mechanical movement, and generators that perform the inverse task of converting mechanical movement into electric current.
The world's first electric motor was created by Faraday, and the principle of its operation remained incomprehensible for a long time, and even now only specialists in unipolar machines understand it. But already the electromagnetic self-rotator of the Hungarian inventor Anjos Jedlik, built by him in 1828, resembles modern collector electric motors, usually operating on direct current. Such a current is given, for example, by galvanic batteries or accumulators.
The principle of operation of the Jedlik self-rotator is to automatically remagnetize an electromagnet in such a way that its poles change places, depending on the position of this electromagnet. Jedlik placed an electromagnet with a core on the tip of a support, like a compass needle, and lowered both ends of its winding into two semicircular cups with mercury, isolated from each other. The positive pole of the battery was connected to one cup, and the negative pole to the other. Cups with mercury played the role of ordinary current collectors, only with much less friction. Above the electromagnet was a winding connected to a current source. In principle, this winding could well be replaced by an ordinary permanent magnet, which we will do for simplicity. It was possible to do without this magnet altogether, bearing in mind that the Earth itself is also a magnet, and that both the compass needle and the electromagnet in its place would be installed in a completely definite position - from one pole to another.
If Jedlik's electromagnet was already in this position when current was applied to it, nothing will change - it will only become even more established in this position, and it will be difficult to move it from its place. But if the electromagnet was in an arbitrary position, then when current is applied to it, it will turn around to take a stable - from pole to pole - position. However, the cups with mercury are located in such a way that, having approached their stable position, the electromagnet turned out to be switched. The ends of the windings jumped into other cups, the poles of the electromagnet changed places, and, having slipped through a stable position by inertia, he again tried to occupy it, but already a new one, at an angle of 180 ° to the previous one. When approaching this new stable position, everything was repeated, and the electromagnet constantly rotated (Fig. 369).
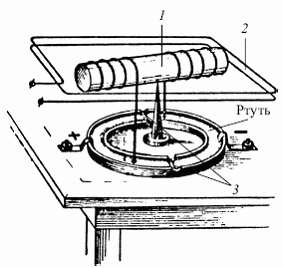
1 - electromagnet; 2 - winding; 3 - isolation
Subsequently, the mercury half-rings were replaced by copper plates, the ends of the windings carried graphite contacts - brushes, but the principle of operation of the electric motor remained the same. Unless the number of poles of a rotating electromagnet - an armature or a rotor - was increased, the number of copper plates at the ends of the windings also increased, and they began to be combined into a collector. Only the most low-power motors for toys or models remained with two plates on the collector. Then the permanent magnets on the stationary part of the electric motor - the stator - were replaced with electromagnets and got almost what we see in electric machines today. On some of the cars direct current, however, permanent magnets remained - where for simplicity, where for efficiency - they do not need to be powered by current (Fig. 370).
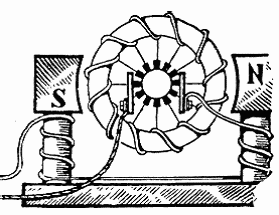
If we apply current to such an electric machine, the rotor or armature starts to rotate, transferring rotation to the shaft. If we ourselves rotate the shaft of the electric machine, we can remove current from the brushes or from the stator windings. Not all electric machines work equally well in both electric motor and generator modes. For example, a car starter for starting an engine is a typical electric motor, but it is no good as a generator. A modern car generator is the same unusable electric motor. But there are electric machines that work equally well as a motor and as a generator, they are called reversible.
Most often, these are DC electric machines. Let's connect a small electric motor with a permanent magnet, at least from a children's electrified toy, to a battery. Its rotor will rotate, doing work, for example, lifting a load. And now we will connect a light bulb from a flashlight to the motor instead of a battery and let the load fall. Falling, the load rotates the rotor of the motor, which has become a generator for a while, and the light bulb lights up (Fig. 371, a). In this experiment, the property of reversibility of electrical machines was manifested. This property is widely used in technology, in particular, in the accumulation and release of energy, its recovery.
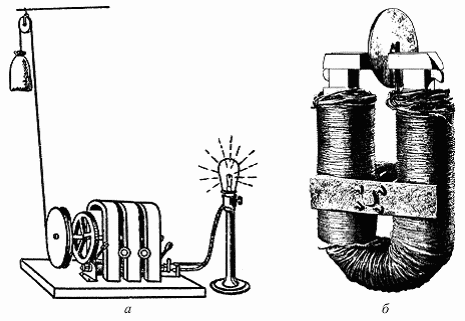
Electric machines, both motors and generators suitable for practical use, appeared immediately after the discovery of Faraday (Fig. 371, b). Moreover, the creation of the first generator of an almost modern type is associated with an event that is more like a legend than a true story. Nevertheless, this case was real.
A few weeks after the discovery of the phenomenon of electromagnetic induction, someone brought to the patent office the design of a generator with permanent magnets, signing only the initials P. M. For that time, this design was unexpected and new. After all, they tried to make the first electric machines look like steam engines - with rocker arms, spools, cranks and connecting rods. But the design of P.M.'s machine, its main features, according to Academician M.P. Kostenko, "... were so correct that for many years they determined the designs of machines of later inventors." Unfortunately, it was not possible to establish the identity of this mysterious P.M.
In 1838, electric motors appeared on the first electric car, which was born much earlier than the first car with an engine internal combustion. It was built by the Englishman R. Davidson in London and tested there.
In the same 1838, the Russian engineer B.S. Yakobi installed an electric motor of his design on a boat 8.5 m long and 2.1 m wide, which accommodated 16 people. The boat made a sensation when tested on the Neva in St. Petersburg, as it could move not only along the river, but also against it. It should not be forgotten that the engine power was only 0.5 kW, negligible by today's standards. The electric boat, like the electric car, was powered by galvanic cells.
Since then, the fundamentals of the design of DC electric machines have not fundamentally changed.
Where does the magnetic field go?
No matter how good DC electric motors were, which, by the way, are still used now in many cases, but not in all. The collector assembly with brushes was very unreliable: it sparked, often failed, and was not cheap. This prompted scientists to look for a way to "brushless" operation of motors and generators, and such a method was found. This helped to make an alternating current and a traveling magnetic field.
At first, when current was obtained from galvanic batteries, alternating current was not heard of. But the famous experiment of Faraday made it possible to obtain exactly an alternating current: when the magnet began to be pushed into the coil, the current arose, and then, when the magnet stopped moving, the current stopped. If you move the magnet back and forth continuously, we get a real alternating current, and without any collectors, directly from the coil. This is good, but there are also inconveniences - it is not very easy to move the magnet back and forth, it is much easier to rotate it (Fig. 372).

And so it was done. They took three coils with cores, placed them in a circle at an angle of 120 °, and inside the circle they began to rotate a magnet - permanent or electric, which, however, gives the same result. When the pole of the magnet approached the coil, a current arose (induced) in it, just as in Faraday's experiment. The magnet could be rotated very quickly, which made it possible to obtain sufficiently large currents. This is how a three-phase alternating current generator was invented - each coil gave its own phase (Fig. 373). The current in these phases increased and decreased alternately, also with a shift of 120°. As for the motor, which can be powered by such a three-phase current, it is fundamentally no different from a generator. The same coils, the same magnet - the rotor. The generator coils are connected by wires (possible for thousands of kilometers!) With the motor coils, and the following happens.
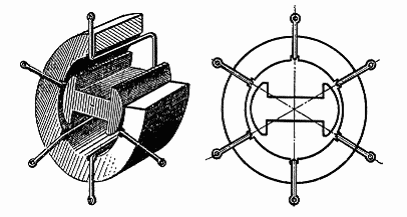
When the pole of the generator magnet passes by any coil, the greatest current arises in it, which magnetizes the corresponding motor coil. It is to this coil that the same pole of the motor magnet tends, and if it is not very disturbed, then it will exactly repeat the rotation of the generator magnet. We got a synchronous motor (motor), i.e. one in which the rotor-magnet moves synchronously with the rotor-magnet of the generator (Fig. 374). In some cases, when, for example, it is necessary to accurately transmit the rotation of the generator shaft to long distance, such a motor is very useful. But most often the rotation of the rotor-magnet encounters great resistance, and it can stop, lose its rhythm.
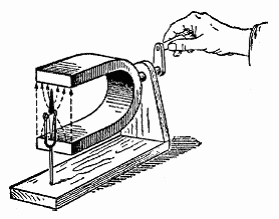
To prevent this from happening, the Russian electrical engineer M. O. Dolivo-Dobrovolsky in 1888 came up with an asynchronous, or, as it is now called, asynchronous motor, where the rotor can lag behind the rotating magnetic field. Imagine that instead of a permanent magnet, the rotor consists of a coil, just like a DC motor, only with a short-circuited collector. In fact, the collector is simply not needed here, and the turns of the coil can be so simplified that they can be made in the form of rods connected by rings at the ends. The design of such a rotor was most widely used and was called short-circuited, since indeed each rod-turn of it is short-circuited (Fig. 375, a). And also because of the striking external resemblance of such a rotor to a squirrel-cage wheel, which also rotates when a squirrel runs along it, the rotor was called that - a squirrel wheel (Fig. 375, b, c, d). These two names have equally taken root in the rotor of an asynchronous machine, which is extremely widespread in technology. Less common are machines where the rotor actually has coil windings.
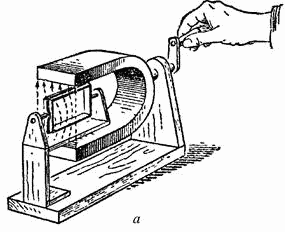
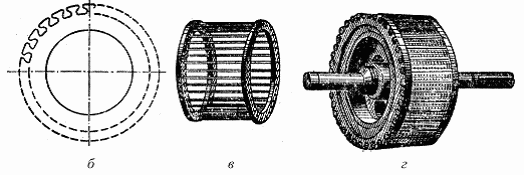
So, the rotating magnetic field of the fixed stator coils of the motor begins to induce electricity in the windings or rods of the fixed rotor, turning them into electromagnets. Those, in turn, behave as a magnet-rotor is supposed to behave - it is carried away by the magnetic field of the stator and begins to rotate.
This is where the difference between synchronous and asynchronous motors is visible. If in the first one the magnet-rotor exactly repeats the rotation of the magnetic field, then in the second such repetition is impossible in principle. If the rotor with the windings begins to rotate at the same speed as the magnetic field, then a moment will come when the current will no longer be induced in the windings, since there will be no relative movement of the magnetic field and the windings. The rotor then, having completely demagnetized, begins to lag behind the rotating magnetic field, but that was not the case. When lagging behind, the relative rotation of the rotor and the field begins again, again the rotor becomes a magnet and again begins to catch up with the magnetic field.
As a result, the rotor of an asynchronous motor always lags behind the rotating magnetic field, and this lag is the greater, the greater the resistance to rotation of the rotor. But in general, this lag is small and for squirrel-cage motors does not exceed a few percent. The network connection diagram and the main details of the asynchronous motor are shown in fig. 376.
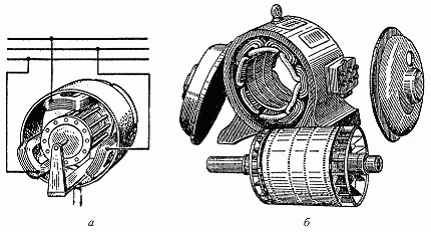
The stator can be both cylindrical and annular. You can "cut" the squirrel wheel, straighten it, placing it along a straight line, just like rails with sleepers. The sleepers in this case will play the role of rods, and the rails - the rings that close them. Let's put a trolley on these rails, in which we will install coils of a cut and straightened stator in the same way. Let's let the current into the stator coils, and we will get not a rotating, but a running magnetic field, which will tend to move forward or backward the sleepers-rods of the straightened rotor. And since it is always more difficult to move the path than to go along this path yourself, the cart with the coils will go along the path, driven by a traveling magnetic field.
Thus, a linear electric motor was created, which has become widespread now and is very promising for the future - after all, all maglev trains are driven precisely by linear electric motors, and this is how it is supposed to be in the future. Linear electric motors move high-speed elevators of skyscrapers, precise mechanisms for moving machine tools, accelerate aircraft for their accelerated launch from aircraft carriers.
Electricity without cars?
It turns out that electric machines are not at all necessary for generating electricity. And, as almost always in electrical engineering, it all started with Faraday ...
One day in 1832, Londoners who found themselves on Waterloo Bridge were interested in an unusual spectacle. A group of people among whom one could see famous physicist Faraday, was engaged in immersing two copper sheets in the Thames water, connected by wires to a galvanometer. The device stood on a table in the middle of the bridge, and next to it was the scientist himself, giving orders to his assistants. Faraday believed that if the waters of a river flowing from west to east cross, at least partially, the Earth's magnetic field, then they are like conductors crossing the magnetic field of a magnet. And in this case, as Faraday himself proved, an electric current arises in the conductor. Copper sheets, between which, as between metal banks, the water of the Thames flowed, had to connect these water conductors with a galvanometer, and transfer the resulting current to it.
However, alas, the experiment failed. Nevertheless, in 1832, when Faraday conceived and substantiated this experiment, with with good reason can be considered the birth year of the magnetohydrodynamic generator. The name of this generator consists of three words - magnet, hydro (water) and dynamics (movement) - and means generating electricity when water moves in a magnetic field.
Why did Faraday's experiment fail? First of all, because the water of the Thames was not such a good conductor of electricity. There were, of course, other reasons - the intensity of the Earth's magnetic field is very low.
But the practical use of magnetohydrodynamic (abbreviated as MHD) generators was still far away.
True, in 1907-1910. the first patents were issued for the use of MHD electricity generation using ionized gas instead of liquid. This is a big step forward, because ionized gas, usually heated to a temperature of 2,500 - 3,000 ° C, is a widespread combustion product of many fuels. Therefore, there was a hope of directly converting the heat of combustion of fuel into electricity, without complex machines with many moving parts. But things did not go beyond patents - scientists did not fully imagine the ongoing processes, and there were no materials capable of operating in a gaseous medium at a temperature of 2,500 - 3,000 ° C.
But in 1944, the Swedish scientist Hannes Alven, studying the behavior of the cosmic plasma filling the interstellar space in a magnetic field, deduced the basic laws of a new science - magnetohydrodynamics. And space science was immediately mastered on Earth and used to create workable MHD generators.
At first glance, these generators are quite simple. Fuel is burned in the combustion chamber, and in a nozzle similar to a rocket, the combustion products (gases), expanding, increase their speed to supersonic. This nozzle is located between the poles of a strong electromagnet, and inside the nozzle, on the path of hot gases, electrodes are installed (Fig. 377).

The magnetic field "sorts" negatively charged electrons and positively charged gas ions, directing them along different trajectories. These streams of charged particles cause the appearance electric charges on the corresponding electrodes, and if they are connected, then an electric current.
Indeed, there are no moving parts in an MHD generator, unless, of course, the gas itself is considered part of the machine. But there are also many bottlenecks.
First of all, a gas heated even up to 2,500 °C is still a low-temperature plasma. It is fully ionized at about four times the temperature. This plasma conducts current a billion times worse than copper, and even worse than water in the English Channel. But this difficulty turned out to be overcome with the help of alkali metal additives, especially potassium. A little, about 1% of potassium is added to the hot gas in the form of its cheap compound - potash, and the electrical conductivity of the plasma is increased by tens of thousands of times.
Further. After all, the walls of the nozzle, and most importantly, the electrode, must work for a long time at 2,500 - 3,000 ° C, and the electrodes, in addition, also conduct electric current well. Materials that can withstand such temperatures for a long time, and even in the presence of aggressive potassium vapor, have not yet been created.
Why are MHD generators so attractive, if they face obvious difficulties in their creation? It turns out that a high ratio useful action. Raise thermal efficiency power plants by at least 1% is a whole event. For more economical operation of heat engines, it is first necessary to increase the temperature of the working fluid: in modern thermal power plants, it is steam. But it is already high - about 700 ° C, and every extra degree is given by desperate labor. Still - the blades and disks of steam turbines, ready to burst from their own rotation, are heated to 700 ° C. From this, their strength does not increase at all. And the creation of even more heat-resistant materials is very, very difficult. Therefore, the maximum efficiency of thermal power plants is now only 45-47%. Increasing the temperature of the working fluid (gas) to 2,500 - 3,000 °C will ensure an increase in efficiency by at least 20%. This is a revolution in energy! There is something to fight for, for the sake of which to create heat-resistant materials for the walls of the nozzle and electrodes!
At the very beginning of the work, it will be useful to give a few definitions and explanations. If, in some place, moving bodies with a charge are affected by a force that does not act on stationary or uncharged bodies, then they say that there is a magnetic field in this place - one of the forms of the more general electromagnetic field. There are bodies that can create a magnetic field around them (and such a body is also affected by the force of the magnetic field), they are said to be magnetized and have a magnetic moment, which determines the property of the body to create a magnetic field. Such bodies are called magnets. It should be noted that different materials react differently to an external magnetic field. There are materials that weaken the action of the external field inside themselves - paramagnets and enhance the external field inside themselves - diamagnets. There are materials with a huge ability (thousands of times) to enhance the external field inside themselves - iron, cobalt, nickel, gadolinium, alloys and compounds of these metals, they are called ferromagnets. Among ferromagnets, there are materials that, after exposure to a sufficiently strong external magnetic field, become magnets themselves - these are magnetically hard materials. There are materials that concentrate an external magnetic field in themselves and, while it acts, behave like magnets; but if the external field disappears, they do not become magnets - these are magnetically soft materials. INTRODUCTION. We are accustomed to the magnet and treat it a little condescendingly as an outdated attribute of school physics lessons, sometimes not even suspecting how many magnets there are around us. There are dozens of magnets in our apartments: in electric shavers, speakers, tape recorders, in watches, in jars of nails, finally. We ourselves are also magnets: the biocurrents flowing in us give rise around us to a bizarre pattern of magnetic lines of force. The earth we live on is a giant blue magnet. The sun is a yellow plasma ball - an even grander magnet. Galaxies and nebulae, barely distinguishable by telescopes, are magnets incomprehensible in size. Thermonuclear fusion, magnetodynamic power generation, acceleration of charged particles in synchrotrons, recovery of sunken ships - all these are areas where grandiose magnets, never seen before in size, are required. The problem of creating strong, superstrong, ultrastrong and even stronger magnetic fields has become one of the main problems in modern physics and technology. The magnet has been known to man since time immemorial. Mentions of magnets and their properties have come down to us in the writings of Thales of Miletus (c. 600 BC) and Plato (427-347 BC). The very word "magnet" arose due to the fact that natural magnets were discovered by the Greeks in Magnesia (Thessaly). Natural (or natural) magnets are found in nature in the form of deposits of magnetic ores. The University of Tartu has the largest known natural magnet. Its mass is 13 kg, and it is able to lift a load of 40 kg. Artificial magnets are magnets created by man on the basis of various ferromagnets. So-called "powder" magnets (made of iron, cobalt and some other additives) can hold a load of more than 5000 times their own weight. There are artificial magnets of two different types : Some are the so-called permanent magnets, made from “hard magnetic” materials. Their magnetic properties are not related to the use of external sources or currents. Another type includes the so-called electromagnets with a core of "soft magnetic" iron. The magnetic fields created by them are mainly due to the fact that an electric current passes through the wire of the winding covering the core. In 1600, a book by the royal physician W. Gilbert “On the magnet, magnetic bodies and the large magnet - the Earth” was published in London. This work was the first attempt known to us to study magnetic phenomena from the standpoint of science. This work contains the then available information about electricity and magnetism, as well as the results of the author's own experiments. From everything that a person encounters, he first of all seeks to derive practical benefit. The magnet did not pass this fate. In my work, I will try to trace how magnets are used by man not for war, but for peaceful purposes, including the use of magnets in biology, medicine, and in everyday life. USE OF MAGNETS. The following is a brief overview of devices and areas of science and technology where magnets are used. KOMPAS, a device for determining horizontal directions on the ground. It is used to determine the direction in which the sea, aircraft, ground vehicle is moving; the direction in which the pedestrian is walking; directions to some object or landmark. Compasses are divided into two main classes: magnetic compasses such as arrows, which are used by topographers and tourists, and non-magnetic, such as a gyrocompass and a radio compass. By the 11th century refers to the message of the Chinese Shen Kua and Chu Yu about the manufacture of compasses from natural magnets and their use in navigation. If a long needle made of a natural magnet is balanced on an axis that allows it to freely rotate in a horizontal plane, then it always faces north with one end and south with the other. By marking the end pointing north, you can use such a compass to determine directions. Magnetic effects were concentrated at the ends of such a needle, and therefore they were called poles (north and south, respectively). The main application of the magnet is in electrical engineering, radio engineering, instrumentation, automation and telemechanics. Here, ferromagnetic materials are used to manufacture magnetic circuits, relays, etc. In 1820, G. Oersted (1777–1851) discovered that a conductor with current acts on a magnetic needle, turning it. Literally a week later, Ampere showed that two parallel conductors with current in the same direction attract each other. Later, he suggested that all magnetic phenomena are due to currents, and the magnetic properties of permanent magnets are associated with currents constantly circulating inside these magnets. This assumption is fully consistent with modern ideas. Electric machine generators and electric motors are rotary type machines that convert either mechanical energy into electrical (generators), or electrical into mechanical (motors). The operation of generators is based on the principle of electromagnetic induction: in a wire moving in a magnetic field, electromotive force(EMF). The action of electric motors is based on the fact that a force acts on a current-carrying wire placed in a transverse magnetic field. Magnetoelectric devices. In such devices, the force of the interaction of the magnetic field with the current in the winding turns of the moving part is used, which tends to turn the last one. Induction electricity meters. An induction meter is nothing more than a low-power AC motor with two windings - a current winding and a voltage winding. A conductive disk placed between the windings rotates under the action of a torque proportional to the power input. This moment is balanced by the currents induced in the disk by the permanent magnet, so that the rotational speed of the disk is proportional to the power consumed. Electric wristwatches are powered by a miniature battery. They require far fewer parts to operate than mechanical watches; for example, a typical electric portable clock has two magnets, two inductors, and a transistor. A lock is a mechanical, electrical or electronic device that limits the possibility of unauthorized use of something. The lock can be actuated by a device (key) held by a certain person, information (digital or alphabetic code) entered by this person, or some individual characteristic (for example, retinal pattern) of this person. The lock usually temporarily connects two nodes or two parts to each other in one device. Most often, locks are mechanical, but electromagnetic locks are increasingly being used. Magnetic locks. Cylinder locks of some models use magnetic elements. The lock and the key are equipped with counter coded sets of permanent magnets. When the correct key is inserted into the keyhole, it attracts and sets the internal magnetic elements of the lock into position, which allows the lock to be opened. Dynamometer - mechanical or electrical appliance to measure the traction force or torque of a machine, machine tool or engine. Brake dynamometers come in a wide variety of designs; these include, for example, the Prony brake, hydraulic and electromagnetic brakes. An electromagnetic dynamometer can be made in the form of a miniature device suitable for measuring the characteristics of small engines. A galvanometer is a sensitive device for measuring weak currents. The galvanometer uses the torque generated by the interaction of a horseshoe-shaped permanent magnet with a small current-carrying coil (weak electromagnet) suspended in the gap between the poles of the magnet. The torque, and hence the deflection of the coil, is proportional to the current and the total magnetic induction in the air gap, so that the scale of the instrument is almost linear with small deflections of the coil. Devices based on it are the most common type of devices. | | | | | | | | The range of manufactured devices is wide and varied: switchboard devices for direct and alternating current (magnetoelectric, magnetoelectric with a rectifier and electromagnetic systems), combined devices, ampere-voltmeters, for diagnosing and adjusting the electrical equipment of cars, measuring the temperature of flat surfaces, devices for equipping school classrooms, testers and meters of various electrical parameters Production of abrasives - small, hard, sharp particles used in free or bound form for mechanical processing (including shaping, peeling, grinding, polishing) of various materials and products from them (from large steel boards to plywood sheets, optical glasses and computer chips). Abrasives are either natural or artificial. The action of abrasives is to remove part of the material from the treated surface. During the production of artificial abrasives, the ferrosilicon present in the mixture settles to the bottom of the furnace, but small amounts of it are embedded in the abrasive and later removed by a magnet. The magnetic properties of matter are widely used in science and technology as a means of studying the structure of various bodies. So the sciences arose: Magnetochemistry (magnetochemistry) - section physical chemistry, which studies the relationship between magnetic and chemical properties substances; in addition, magnetochemistry investigates the influence of magnetic fields on chemical processes. magnetochemistry is based on modern physics of magnetic phenomena. The study of the relationship between magnetic and chemical properties makes it possible to elucidate the features of the chemical structure of a substance. Magnetic flaw detection, a method for searching for defects based on the study of magnetic field distortions that occur at the sites of defects in products made of ferromagnetic materials. . Microwave technology electromagnetic radiation (100h300 000 million hertz), located in the spectrum between ultra-high television frequencies and far infrared frequencies Communication. Microwave radio waves are widely used in communications technology. In addition to various military radio systems, there are numerous commercial microwave links in all countries of the world. Since such radio waves do not follow the curvature of the earth's surface, but propagate in a straight line, these communication links usually consist of relay stations installed on hilltops or on radio towers at intervals of about 50 km. Heat treatment of food products. Microwave radiation is used for heat treatment of food products at home and in the food industry. The energy generated by powerful vacuum tubes can be concentrated in a small volume for highly efficient cooking of products in the so-called. microwave or microwave ovens, characterized by cleanliness, noiselessness and compactness. Such devices are used in aircraft galleys, railway dining cars and vending machines where fast food preparation and cooking is required. The industry also produces household microwave ovens. The rapid progress in the field of microwave technology is largely associated with the invention of special electrovacuum devices - the magnetron and the klystron, capable of generating large amounts of microwave energy. An oscillator based on a conventional vacuum triode, used at low frequencies, turns out to be very inefficient in the microwave range. Magnetron. In the magnetron, invented in Great Britain before the Second World War, these shortcomings are absent, since a completely different approach to the generation of microwave radiation is taken as a basis - the principle of a cavity resonator. The magnetron has several cavity cavities symmetrically located around the cathode located in the center. The instrument is placed between the poles of a strong magnet. Traveling wave lamp (TWT). Another electrovacuum device for generating and amplifying electromagnetic waves in the microwave range is a traveling wave lamp. It is a thin evacuated tube inserted into a focusing magnetic coil. Particle accelerator, a device in which directed beams of electrons, protons, ions and other charged particles with an energy much higher than thermal energy are obtained using electric and magnetic fields. Numerous and diverse types of equipment are used in modern accelerators, incl. powerful precision magnets. Accelerators play an important practical role in medical therapy and diagnostics. Many hospitals around the world today have at their disposal small electron linear accelerators that generate intense x-rays used for tumor therapy. To a lesser extent, cyclotrons or synchrotrons generating proton beams are used. The advantage of protons in tumor therapy over X-rays is a more localized energy release. Therefore, proton therapy is especially effective in the treatment of brain and eye tumors, when damage to surrounding healthy tissues should be as minimal as possible. Representatives of various sciences take into account magnetic fields in their research. A physicist measures the magnetic fields of atoms and elementary particles, an astronomer is studying the role of cosmic fields in the formation of new stars, a geologist is looking for deposits of magnetic ores by the anomalies of the Earth's magnetic field, and recently biology has also been actively involved in the study and use of magnets. biological science of the first half of the 20th century confidently described vital functions, not at all taking into account the existence of any magnetic fields. Moreover, some biologists considered it necessary to emphasize that even a strong artificial magnetic field does not have any effect on biological objects. In encyclopedias, nothing was said about the influence of magnetic fields on biological processes. AT scientific literature all over the world every year there were single positive considerations about this or that biological effect of magnetic fields. However, this weak brook could not melt the iceberg of distrust even in the formulation of the problem itself... And suddenly the brook turned into a turbulent stream. The avalanche of magnetobiological publications, as if breaking off from some peak, has been constantly increasing since the beginning of the 60s and drowning out skeptical statements. From the alchemists of the 16th century to the present day, the biological action of the magnet has found admirers and critics many times. Repeatedly over the course of several centuries, surges and recessions of interest in the therapeutic effect of the magnet were observed. With its help, they tried to treat (and not unsuccessfully) nervous diseases, toothache, insomnia, pain in the liver and stomach - hundreds of diseases. For medicinal purposes, the magnet began to be used, probably earlier than for determining the cardinal points. As a local external remedy and as an amulet, the magnet was very popular with the Chinese, Hindus, Egyptians, and Arabs. GREEKS, Romans, etc. Its healing properties are mentioned in their writings by the philosopher Aristotle and the historian Pliny. In the second half of the 20th century, magnetic bracelets became widespread, having a beneficial effect on patients with blood pressure disorders (hypertension and hypotension). In addition to permanent magnets, electromagnets are also used. They are also used for a wide range of problems in science, technology, electronics, medicine (nervous diseases, vascular diseases of the extremities, cardiovascular diseases, cancers). Most of all, scientists tend to think that magnetic fields increase the body's resistance. There are electromagnetic blood velocity meters, miniature capsules that, using external magnetic fields, can be moved through blood vessels to expand them, take samples at certain sections of the path, or, conversely, locally remove various medicines from the capsules. The magnetic method of removing metal particles from the eye is widely used. Most of us are familiar with the study of the work of the heart with the help of electrical sensors - an electrocardiogram. The electrical impulses produced by the heart create a magnetic field of the heart, which in max values is 10-6 of the Earth's magnetic field strength. The value of magnetocardiography is that it provides information about the electrically "silent" areas of the heart. It should be noted that biologists are now asking physicists to give a theory of the primary mechanism of the biological action of the magnetic field, and physicists in response demand more verified biological facts from biologists. It is obvious that close cooperation of various specialists will be successful. An important link uniting magnetobiological problems is the response of the nervous system to magnetic fields. The brain is the first to react to any changes in external environment. It is the study of its reactions that will be the key to solving many problems of magnetobiology. The simplest conclusion that can be drawn from the above is that there is no area of applied human activity where magnets would not be used. References: 1) TSB, second edition, Moscow, 1957. 2) Kholodov Yu.A. “Man in the Magnetic Web”, “Knowledge”, Moscow, 1972 3) Materials from the Internet Encyclopedia 4) Putilov K.A. "Physics course", "Physmatgiz", Moscow, 1964.
Back forward
Attention! The slide preview is for informational purposes only and may not represent the full extent of the presentation. If you are interested this work please download the full version.
Type of. Explanation of new material.
Goals.
- Educational. Give the concept of a permanent magnet, the Earth's magnetic field; explore the interaction of the poles of two magnets, get acquainted with the properties of the magnetic field; to form the ability to apply the acquired knowledge to solve problems and perform practical tasks, expand the horizons of students in the field of magnetic phenomena.
- Educational. Learn to evaluate your work objectively.
- Developing. The development of students' voluntary attention, thinking (the ability to analyze, compare, build analogies, draw conclusions.), Cognitive interest (based on a physical experiment); formation of worldview concepts about the cognizability of the world.
DURING THE CLASSES
Hello, have a seat.
Guys, let's remember what you know about the electric field.
Independent work
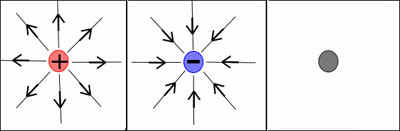
Let's check the work and rate ourselves.
Well done! Today we will dive into the world of the science of magnetism, research, interesting facts related to magnetism.
Write in your notebook the date and the topic of the lesson “Permanent magnets. Earth's magnetic field”.
The history of the magnet has more than two and a half thousand years. In the VI century. BC e. Ancient Chinese scientists discovered a mineral that can attract iron objects to itself. The Chinese called it “chu-shih”, which means “loving stone”.
The name "magnet" was coined by the ancient Greek playwright Euripides (V century BC), who described its properties in one of his works: it was many centuries ago. In search of a sheep, the shepherd went to unfamiliar places, to the mountains. There were black stones all around. He noticed with amazement that his iron-tipped stick was being pulled towards the stones, as if some invisible hand was grasping and holding it. Struck by the miraculous power of the stones, the shepherd brought them to the nearest city - Magnes. Hence the name of these stones. Magnet means "stone from Magnesia".
Bodies that remain magnetized for a long time are called permanent magnets or simply magnets.
Magnets come in different shapes: strip, arcuate, ring. Every magnet, like a magnetic needle, must have two poles: northern(N) and southern(S).
(demonstration of a magnetic needle, strip and arc magnets)
And now you guys, in the course of performing experimental tasks, will explore some properties of magnets. The cutlery is already on your tables. Performing tasks, you will draw the appropriate conclusions.
Exercise 1.
Equipment: metal clips, strip magnets.
Take a strip magnet, bring it first to the paper clips, and then to the copper screw. What are you observing? Make a conclusion. ( Students conclude Not all substances are attracted by magnets.)
Task 2.
Take a strip magnet, bring a few paper clips exactly to the middle of the magnet, where the border between the red and blue halves passes. Does a magnet attract paperclips?
Move the paperclips to different places on the magnet, starting from the middle. What places show the strongest magnetic action? Repeat the same with the arc magnet.
Make a conclusion. ( Students conclude- there are areas with stronger magnetic properties)
Natural magnets are found in nature - iron ore (the so-called magnetic iron ore). It is now known that natural magnets are pieces of magnetic iron ore (magnetite), consisting of FeO (31%) and Fe 2 O 3 (69%). There are rich deposits of magnetic iron ore in the Urals, in the Ukraine, in Karelia, the Kursk region, and in many other places.
In ancient times, they tried to explain the properties of a magnet by attributing a “living soul” to it. The magnet, according to the ideas of ancient people, "rushed" to the iron for the same reason as the dog to the piece of meat.
Task 3.
Take a strip magnet, bring some paper clips near the magnet, but don't touch them. What are you observing? Make a conclusion. ( Students conclude that interaction occurs at a distance)
Now we know that it's all about the special field created by the magnet. Every magnet has a magnetic field around it.. This field attracts the iron to the magnet.
The magnetic field is a special kind of matter that differs from matter and exists around magnetized bodies.
Magnetite has not very strong magnetic properties. At present, it is possible to create artificial magnets with a much stronger magnetic field. The material for them are alloys based on iron, nickel, cobalt and some other metals. In an external magnetic field, they are magnetized, after which they can be used as independent permanent magnets.
Task 4.
Equipment: iron nail, paper clips, magnet.
Take a nail and bring it to the paperclips. Do staples stick to nails?
Rub the nail against the magnet in one direction and then bring it up to the paperclips. Do staples stick?
Make a conclusion. ( Students draw conclusions- the nail acquired the properties of a permanent magnet)
Conclusion. Iron, steel, nickel, cobalt and some other alloys acquire magnetic properties in the presence of magnetic iron ore.
Video “Ferromagnets” (Presentation)
If a magnetic needle is brought close to another similar arrow, then they will turn and set opposite each other with opposite poles ( show by experience).
Equipment: magnet and magnetic needle.
Bring a magnet to the blue and then to the red end of the magnetic needle. What can be said about the interaction of a magnetic needle and a magnet?
In which case the magnetic needle is attracted, and in which case it is repelled.
Conclusion. Like poles of a magnet and a magnetic needle repel, opposite poles attract.
Video “Interaction of magnets” (Presentation)
The same pattern is true for any magnets: opposite poles attract, like poles repel.
(Solve No. 103 textbook “Physics 9” by S.V. Gromov, N.A. Rodina)
A magnet made in the form of an arrow is used in compasses. The compass was invented about two thousand years ago in China. It was called the sign of the south
Demonstration of the compass, determining the cardinal points
The earth is also a magnet. It has its own magnetic poles and its own magnetic field. That is what makes the compass needle orient all the time in a certain direction.
It is not difficult to understand where exactly it should point: after all, opposite poles attract. Consequently, the north pole of the arrow indicates the direction of the south magnetic pole of the earth. This pole is located in the north of the globe, somewhat away from the North Geographic Pole (on the island of Prince of Wales).
Video “Magnetic poles of the Earth” (Presentation)
Read the last paragraph on page 57 of the textbook and talk about the use of permanent magnets.
Answer the questions:
1. What does the word “magnet” mean?
2. What are natural magnets?
3. How are artificial magnets obtained?
4. What are called magnetic poles?
5. How do the poles of magnets interact with each other?
6. How can you determine the poles of a magnetized steel rod using a magnetic needle?
7. Under the influence of what does the compass needle orient in a certain direction? Where does she point?
8. Where are magnets used?
9. Why does the magnetic iron ore that exists in nature turn out to be magnetized? What magnetized him?
10. What is called a magnetic field?
1. How will the magnets interact?
2. Mark the geographic and magnetic poles of the Earth in the figure.
3. No. 1472, 1473, 1474, 1475, 1476 (“Collection of problems in physics 7-9” V.I. Lukashik, E.V. Ivanova)
Well done guys, great job! Let's write down our homework.
Paragraph 21 No. 104
Methodological support
- “Physics 9” S.V. Gromov, N.A. motherland
- “Collection of problems in physics 7-9” V.I.Lukashik, E.V. Ivanova
- Video clips, drawings, which are in the public domain of the Internet
Natural and artificial magnets
Among the iron ores mined for the metallurgical industry, there is an ore called magnetic iron ore. This ore has the ability to attract iron objects to itself.
A piece like this iron ore called natural magnet, and the property of attraction it manifests is magnetism.
Nowadays, the phenomenon of magnetism is used extremely widely in various electrical installations. However, now they use not natural, but the so-called artificial magnets.
Artificial magnets are made from special grades of steel. A piece of such steel is magnetized in a special way, after which it acquires magnetic properties, that is, it becomes.
The form of permanent magnets can be the most diverse, depending on their purpose.
In a permanent magnet, only its poles have the forces of attraction. The end of the magnet, facing north, agreed to be called the north pole of a magnet, and the end facing south - the south pole of a magnet. Each permanent magnet has two poles: north and south. The north pole of a magnet is denoted by the letter C or N, the south pole by the letter Y or S.
The magnet attracts iron, steel, cast iron, nickel, cobalt. All these bodies are called magnetic bodies. All other bodies that are not attracted to a magnet are called non-magnetic bodies.
The structure of a magnet. Magnetization
Any body, including magnetic, consists of the smallest particles - molecules. Unlike the molecules of non-magnetic bodies, the molecules of a magnetic body have magnetic properties, representing molecular magnets. Inside the magnetic body, these molecular magnets are located with their axes in different directions, as a result of which the body itself does not exhibit any magnetic properties. But if these magnets are forced to turn around their axes so that their north poles are turned in one direction and their south poles in the other, then the body will acquire magnetic properties, i.e., it will become a magnet.
The process by which a magnetic body acquires the properties of a magnet is called magnetization. In the manufacture of permanent magnets, magnetization is carried out using an electric current. But you can magnetize the body in another way, using an ordinary permanent magnet.
If a rectilinear magnet is sawn along a neutral line, then two independent magnets will be obtained, and the polarity of the ends of the magnet will be preserved, and opposite poles will appear at the ends obtained as a result of sawing.
Each of the resulting magnets can also be divided into two magnets, and no matter how much we continue this division, we will always get independent magnets with two poles. It is impossible to get a bar with one magnetic pole. This example confirms the position that the magnetic body consists of many molecular magnets.
Magnetic bodies differ from one another in the degree of mobility of molecular magnets. There are bodies that quickly magnetize and just as quickly demagnetize. And, conversely, there are bodies that are magnetized slowly, but retain their magnetic properties for a long time.
So iron is quickly magnetized under the action of a foreign magnet, but it is also quickly demagnetized, that is, it loses its magnetic properties when the magnet is removed. Steel, having been magnetized once, retains its magnetic properties for a long time, that is, it becomes a permanent magnet.
The property of iron to quickly magnetize and demagnetize is explained by the fact that the molecular magnets of iron are extremely mobile, they easily turn under the action of external magnetic forces, but just as quickly return to their former disorderly position when the magnetizing body is removed.
However, in the gland, a small part of the magnets, even after the removal of the permanent magnet, still continues to remain for some time in the position that they assumed during magnetization. Consequently, iron after magnetization retains very weak magnetic properties. This is confirmed by the fact that when the iron plate was removed from the pole of the magnet, not all the filings fell from its end - a small part of them remained still attracted to the plate.
The property of steel to remain magnetized for a long time is explained by the fact that the molecular magnets of steel hardly turn in the right direction during magnetization, but they retain their steady position for a long time even after the removal of the magnetizing body.
The ability of a magnetic body to exhibit magnetic properties after magnetization is called residual magnetism.
The phenomenon of residual magnetism is caused by the fact that the so-called retarding force acts in the magnetic body, which keeps the molecular magnets in the position occupied by them during magnetization.
In iron, the action of the retarding force is very weak, as a result of which it quickly demagnetizes and has a very small residual magnetism.
The property of iron to quickly magnetize and demagnetize is extremely widely used in electrical engineering. Suffice it to say that the cores of all those used in electrical apparatus are made from special iron, which has extremely low residual magnetism.
Steel has a large holding power, so that it retains the property of magnetism. Therefore, they are made from special steel alloys.
The properties of a permanent magnet are adversely affected by impacts, shocks and sudden temperature fluctuations. If, for example, a permanent magnet is heated red-hot and then allowed to cool, it will completely lose its magnetic properties. Similarly, if a permanent magnet is subjected to shocks, then its attractive force will noticeably decrease.
This is explained by the fact that with strong heating or impacts, the action of the retarding force is overcome and thereby the ordered arrangement of molecular magnets is disturbed. This is why permanent magnets and devices that have permanent magnets must be handled with care.
Around any magnet there is a so-called.
A magnetic field is a space in which magnetic forces act. The magnetic field of a permanent magnet is that part of the space in which the fields of a rectilinear magnet act and the magnetic forces of this magnet.
The magnetic forces of a magnetic field act in certain directions. The directions of action of magnetic forces are agreed to be called magnetic field lines. This term is widely used in the study of electrical engineering, but we must remember that magnetic lines of force are not material: this is a conditional concept introduced only to facilitate understanding of the properties of a magnetic field.
Magnetic field shape, i.e., the location in space of magnetic field lines depends on the shape of the magnet itself.
Magnetic field lines have a number of properties: they are always closed, never intersect, tend to take the shortest path and repel each other if directed in the same direction. It is generally accepted that the lines of force come out of the north pole of the magnet and enter its south pole; inside the magnet, they have a direction from the south pole to the north.
Like magnetic poles repel, opposite magnetic poles attract.
It is easy to verify the correctness of both conclusions in practice. Take a compass and bring one of the poles of a rectilinear magnet to it, for example, the north one. You will see that the arrow will instantly turn its southern end towards the north pole of the magnet. If you quickly turn the magnet 180 °, then the magnetic needle will immediately turn 180 °, i.e. its northern end will be facing south pole magnet.
Magnetic induction. magnetic flux
The force of influence (attraction) of a permanent magnet on a magnetic body decreases with increasing distance between the pole of the magnet and this body. The magnet exhibits the greatest force of attraction directly at its poles, i.e., just where the magnetic lines of force are most densely located. As you move away from the pole, the density of the lines of force decreases, they are located less and less, along with this, the force of attraction of the magnet also weakens.
Thus, the force of attraction of a magnet in different points magnetic field is not the same and is characterized by the density of field lines. To characterize the magnetic field at its various points, a quantity called magnetic field induction.
The magnetic induction of the field is numerically equal to the number of field lines passing through a 1 cm2 area located perpendicular to their direction.
This means that the greater the density of field lines at a given point of the field, the greater the magnetic induction at this point.
The total number of magnetic lines of force passing through any area is called magnetic flux.
The magnetic flux is denoted by the letter F and is associated with magnetic induction by the following relationship:
F = BS,
where Ф - magnetic flux, В - magnetic field induction; S is the area penetrated by the given magnetic flux.
This formula is valid only if the area S is located perpendicular to the direction of the magnetic flux. Otherwise, the magnitude of the magnetic flux will also depend on the angle at which the area S is located, and then the formula will take on a more complex form.
The magnetic flux of a permanent magnet is determined by the total number of lines of force passing through the cross section of the magnet. The greater the magnetic flux of a permanent magnet, the greater the force of attraction this magnet has.
The magnetic flux of a permanent magnet depends on the quality of the steel from which the magnet is made, on the dimensions of the magnet itself and on the degree of its magnetization.
Magnetic permeability
The property of a body to pass a magnetic flux through itself is called magnetic permeability. magnetic flux it is easier to pass through air than through a non-magnetic body.
To be able to compare various substances according to them, it is customary to consider the magnetic permeability of air equal to unity.
Substances whose magnetic permeability is less than one are called diamagnetic. These include copper, lead, silver, etc.
Aluminum, platinum, tin, etc. have a magnetic permeability slightly greater than unity and are called paramagnetic substances.
Substances magnetic permeability which is much more than one (measured in thousands) are called ferromagnetic. These include nickel, cobalt, steel, iron, etc. All kinds of magnetic and electromagnetic devices and parts of various electrical machines are made from these substances and their alloys.
Of practical interest for communication technology are special alloys of iron and nickel, called permalloys.
Meals at school should be well organized. The student must be provided with lunch and a hot breakfast in the dining room. The interval between the first and second meals should not exceed four hours. The best option should be the breakfast of the child at home, while at school he eats a second breakfast
A certain relationship has been established between children's aggression and difficulties in the learning process. Every student wants to have many friends at school, to have good academic performance and good grades. When the child does not succeed, he does aggressive acts. Each behavior is aimed at something, has a semantic
In any Olympiads and various competitions, the child, first of all, expresses himself and fulfills himself. Parents must definitely support their child if he is passionate about intellectual competitions. It is important for a child to realize himself as a part of a society of intellectuals, in which competitive moods reign, and the child compares his achievements
A picky child may not like school food. Often, this is the most common reason for a student to refuse food. Everything comes from the fact that the menu at the school does not take into account the taste needs of each individual child. At school, no one will exclude any food from the diet of an individual child in order to
In order to understand how parents relate to school, it is important to first characterize modern parents, whose age category is very diverse. Despite this, most of them are parents who belong to the generation of the nineties, which differ hard time for the entire population.
The first school fees will forever remain in the memory of each of us. Parents begin to purchase all the necessary stationery starting in August. The main school attribute is the form of the student. The outfit must be carefully selected so that the first grader feels confident. Introduction school uniform justified by many reasons.
Dear pupils and students!
Already now on the site you can use more than 20,000 abstracts, reports, cheat sheets, term papers and theses. Send us your new papers and we will publish them without fail. Let's continue building our abstract collection together!!!
You agree to submit your abstract (diploma, coursework etc.?
Thank you for your contribution to the collection!Application of magnets
Date added: March 2006
At the very beginning of the work, it will be useful to give a few definitions and explanations. If, in some place, moving bodies with a charge are affected by a force that does not act on stationary or uncharged bodies, then they say that in this place there is a magnetic field - one of the forms of the more general electromagnetic field.
There are bodies that can create a magnetic field around them (and such a body is also affected by the force of the magnetic field), they are said to be magnetized and have a magnetic moment, which determines the property of the body to create a magnetic field. Such bodies are called magnets.
It should be noted that different materials react differently to an external magnetic field.
There are materials that weaken the action of the external field inside themselves - paramagnets and enhance the external field inside themselves - diamagnets. There are materials with a huge ability (thousands of times) to enhance the external field inside themselves - iron, cobalt, nickel, gadolinium, alloys and compounds of these metals, they are called ferromagnets.
Among ferromagnets, there are materials that, after exposure to a sufficiently strong external magnetic field, become magnets themselves - these are magnetically hard materials. There are materials that concentrate an external magnetic field in themselves and, while it acts, behave like magnets; but if the external field disappears, they do not become magnets - these are magnetically soft materials
INTRODUCTION
We are accustomed to the magnet and treat it a little condescendingly as an outdated attribute of school physics lessons, sometimes not even suspecting how many magnets there are around us. There are dozens of magnets in our apartments: in electric shavers, speakers, tape recorders, in watches, in jars of nails, finally. We ourselves are also magnets: the biocurrents flowing in us give rise around us to a bizarre pattern of magnetic lines of force. The earth we live on is a giant blue magnet. The Sun is a yellow plasma ball, an even grander magnet. Galaxies and nebulae, barely distinguishable by telescopes, are magnets incomprehensible in size. Thermonuclear fusion, magnetodynamic power generation, the acceleration of charged particles in synchrotrons, the recovery of sunken ships - all these are areas where grandiose, never-before-seen in size magnets are required. The problem of creating strong, superstrong, ultrastrong and even stronger magnetic fields has become one of the main problems in modern physics and technology.
The magnet has been known to man since time immemorial. Mentions of magnets and their properties have come down to us in the writings of Thales of Miletus (approx. 600 BC) and Plato (427-347 BC). The very word "magnet" arose due to the fact that natural magnets were discovered by the Greeks in Magnesia (Thessaly).
Natural (or natural) magnets are found in nature in the form of deposits of magnetic ores. The University of Tartu has the largest known natural magnet. Its mass is 13 kg, and it is able to lift a load of 40 kg.
Artificial magnets are magnets created by man on the basis of various ferromagnets. So-called "powder" magnets (made of iron, cobalt and some other additives) can hold a load of more than 5000 times their own weight.
There are two different types of artificial magnets:
Some are the so-called permanent magnets, made from "hard magnetic" materials. Their magnetic properties are not related to the use of external sources or currents.
Another type includes the so-called electromagnets with a core of "soft magnetic" iron. The magnetic fields created by them are mainly due to the fact that an electric current passes through the wire of the winding covering the core. In 1600, a book by the royal physician W. Gilbert “On the magnet, magnetic bodies and the large magnet - the Earth” was published in London. This work was the first attempt known to us to study magnetic phenomena from the standpoint of science. This work contains the then available information about electricity and magnetism, as well as the results of the author's own experiments.
From everything that a person encounters, he first of all seeks to derive practical benefit. Did not pass this fate and the magnet
In my work, I will try to trace how magnets are used by people not for war, but for peaceful purposes, including the use of magnets in biology, medicine, and in everyday life.
KOMPAS, a device for determining horizontal directions on the ground. It is used to determine the direction in which the sea, aircraft, ground vehicle is moving; the direction in which the pedestrian is walking; directions to some object or landmark. Compasses are divided into two main classes: magnetic compasses such as arrows, which are used by topographers and tourists, and non-magnetic, such as a gyrocompass and a radio compass.
By the 11th century refers to the message of the Chinese Shen Kua and Chu Yu about the manufacture of compasses from natural magnets and their use in navigation. If a
a long needle made of a natural magnet is balanced on an axis that allows it to freely rotate in a horizontal plane, then it always faces north with one end and south with the other. By marking the end pointing north, you can use such a compass to determine directions.
Magnetic effects were concentrated at the ends of such a needle, and therefore they were called poles (north and south, respectively).
The main application of the magnet is in electrical engineering, radio engineering, instrumentation, automation and telemechanics. Here, ferromagnetic materials are used to manufacture magnetic circuits, relays, etc.
In 1820, G. Oersted (1777–1851) discovered that a conductor with current acts on a magnetic needle, turning it. Literally a week later, Ampere showed that two parallel conductors with current in the same direction attract each other. Later, he suggested that all magnetic phenomena are due to currents, and the magnetic properties of permanent magnets are associated with currents constantly circulating inside these magnets. This assumption is fully consistent with modern ideas.
Electric machine generators and electric motors are rotary type machines that convert either mechanical energy into electrical energy (generators) or electrical energy into mechanical energy (motors). The operation of generators is based on the principle of electromagnetic induction: an electromotive force (EMF) is induced in a wire moving in a magnetic field. The action of electric motors is based on the fact that a force acts on a current-carrying wire placed in a transverse magnetic field.
Magnetoelectric devices. In such devices, the force of the interaction of the magnetic field with the current in the winding turns of the moving part is used, which tends to turn the last one. Induction electricity meters. An induction meter is nothing more than a low-power AC motor with two windings - current and voltage. A conductive disk placed between the windings rotates under the action of a torque proportional to the power input. This moment is balanced by the currents induced in the disk by the permanent magnet, so that the rotational speed of the disk is proportional to the power consumed.
Electric wristwatches are powered by a miniature battery. They require far fewer parts to operate than mechanical watches; for example, a typical electric portable clock has two magnets, two inductors, and a transistor. A lock is a mechanical, electrical or electronic device that limits the possibility of unauthorized use of something. The lock can be actuated by a device (key) held by a certain person, information (digital or alphabetic code) entered by this person, or some individual characteristic (for example, retinal pattern) of this person. The lock usually temporarily connects two nodes or two parts to each other in one device. Most often, locks are mechanical, but electromagnetic locks are increasingly being used.
Magnetic locks. Cylinder locks of some models use magnetic elements. The lock and the key are equipped with counter coded sets of permanent magnets. When the correct key is inserted into the keyhole, it attracts and sets the internal magnetic elements of the lock into position, which allows the lock to be opened.
A dynamometer is a mechanical or electrical instrument for measuring the traction force or torque of a machine, machine tool or engine.
Brake dynamometers come in a wide variety of designs; these include, for example, the Prony brake, hydraulic and electromagnetic brakes.
An electromagnetic dynamometer can be made in the form of a miniature device suitable for measuring the characteristics of small engines.
A galvanometer is a sensitive device for measuring weak currents. The galvanometer uses the torque generated by the interaction of a horseshoe-shaped permanent magnet with a small current-carrying coil (weak electromagnet) suspended in the gap between the poles of the magnet. The torque, and hence the deflection of the coil, is proportional to the current and the total magnetic induction in the air gap, so that the scale of the instrument is almost linear with small deflections of the coil. Devices based on it are the most common type of devices.
The range of manufactured devices is wide and varied: switchboard devices for direct and alternating current (magnetoelectric, magnetoelectric with a rectifier and electromagnetic systems), combined devices, ampere-voltmeters, for diagnosing and adjusting the electrical equipment of cars, measuring the temperature of flat surfaces, devices for equipping school classrooms, testers and meters of various electrical parameters
Production of abrasives - small, hard, sharp particles used in free or bound form for mechanical processing (including shaping, peeling, grinding, polishing) of various materials and products from them (from large steel plates to plywood sheets, optical glasses and computer chips). Abrasives are either natural or artificial. The action of abrasives is to remove part of the material from the treated surface. During the production of artificial abrasives, the ferrosilicon present in the mixture settles to the bottom of the furnace, but small amounts of it are embedded in the abrasive and later removed by a magnet.
The magnetic properties of matter are widely used in science and technology as a means of studying the structure of various bodies. This is how the sciences arose:
Magnetochemistry (magnetochemistry) - a branch of physical chemistry that studies the relationship between the magnetic and chemical properties of substances; in addition, magnetochemistry investigates the effect of magnetic fields on chemical processes. magnetochemistry is based on modern physics of magnetic phenomena. The study of the relationship between magnetic and chemical properties makes it possible to elucidate the features of the chemical structure of a substance.
Magnetic flaw detection, a method for searching for defects based on the study of magnetic field distortions that occur at the sites of defects in products made of ferromagnetic materials.
Microwave technology
Super high frequency range (SHF) - the frequency range of electromagnetic radiation (100-300,000 million hertz), located in the spectrum between ultra-high television frequencies and frequencies of the far infrared region
Connection. Microwave radio waves are widely used in communications technology. In addition to various military radio systems, there are numerous commercial microwave links in all countries of the world. Since such radio waves do not follow the curvature of the earth's surface, but propagate in a straight line, these communication links usually consist of relay stations installed on hilltops or on radio towers at intervals of about 50 km.
Heat treatment of food products. Microwave radiation is used for heat treatment of food products at home and in the food industry. The energy generated by powerful vacuum tubes can be concentrated in a small volume for highly efficient cooking of products in the so-called. microwave or microwave ovens, characterized by cleanliness, noiselessness and compactness. Such devices are used in aircraft galleys, railway dining cars and vending machines where fast food preparation and cooking is required. The industry also produces household microwave ovens. The rapid progress in the field of microwave technology is largely associated with the invention of special electrovacuum devices - the magnetron and the klystron, capable of generating large amounts of microwave energy. An oscillator based on a conventional vacuum triode, used at low frequencies, turns out to be very inefficient in the microwave range.
Magnetron. In the magnetron, invented in Great Britain before the Second World War, these shortcomings are absent, since a completely different approach to the generation of microwave radiation is taken as the basis - the principle of a cavity resonator
The magnetron has several cavity resonators arranged symmetrically around the cathode located in the center. The instrument is placed between the poles of a strong magnet.
Traveling wave lamp (TWT). Another electrovacuum device for generating and amplifying electromagnetic waves in the microwave range is a traveling wave lamp. It is a thin evacuated tube inserted into a focusing magnetic coil.
Particle accelerator, a device in which directed beams of electrons, protons, ions and other charged particles with an energy much higher than thermal energy are obtained using electric and magnetic fields.
Modern accelerators use numerous and varied types of technology, including powerful precision magnets.
Accelerators play an important practical role in medical therapy and diagnostics. Many hospitals around the world today have at their disposal small electron linear accelerators that generate intense x-rays used for tumor therapy. To a lesser extent, cyclotrons or synchrotrons generating proton beams are used. The advantage of protons in tumor therapy over X-rays is a more localized energy release. Therefore, proton therapy is especially effective in the treatment of brain and eye tumors, when damage to surrounding healthy tissues should be as minimal as possible.
Representatives of various sciences take into account magnetic fields in their research. A physicist measures the magnetic fields of atoms and elementary particles, an astronomer studies the role of cosmic fields in the process of the formation of new stars, a geologist uses the anomalies of the Earth's magnetic field to find deposits of magnetic ores, and recently biology has also been actively involved in the study and use of magnets.
The biological science of the first half of the 20th century confidently described vital functions, not at all taking into account the existence of any magnetic fields. Moreover, some biologists considered it necessary to emphasize that even a strong artificial magnetic field does not have any effect on biological objects.
In encyclopedias, nothing was said about the influence of magnetic fields on biological processes. In the scientific literature of the whole world, single positive considerations about one or another biological effect of magnetic fields appeared every year. However, this weak brook could not melt the iceberg of distrust even in the formulation of the problem itself... And suddenly the brook turned into a turbulent stream. The avalanche of magnetobiological publications, as if breaking off from some kind of peak, has been constantly increasing since the beginning of the 60s and drowning out skeptical statements.
From the alchemists of the 16th century to the present day, the biological action of the magnet has found admirers and critics many times. Repeatedly over the course of several centuries, surges and recessions of interest in the therapeutic effect of the magnet were observed. With its help, they tried to treat (and not unsuccessfully) nervous diseases, toothache, insomnia, pain in the liver and stomach - hundreds of diseases.
For medicinal purposes, the magnet began to be used, probably earlier than for determining the cardinal points.
As a local external remedy and as an amulet, the magnet was very popular with the Chinese, Hindus, Egyptians, and Arabs. GREEKS, Romans, etc. Its healing properties are mentioned in their writings by the philosopher Aristotle and the historian Pliny.
In the second half of the 20th century, magnetic bracelets became widespread, having a beneficial effect on patients with blood pressure disorders (hypertension and hypotension).
In addition to permanent magnets, electromagnets are also used. They are also used for a wide range of problems in science, technology, electronics, medicine (nervous diseases, vascular diseases of the extremities, cardiovascular diseases, cancers).
Most of all, scientists tend to think that magnetic fields increase the body's resistance.
There are electromagnetic blood velocity meters, miniature capsules that, using external magnetic fields, can be moved through blood vessels to expand them, take samples at certain sections of the path, or, conversely, locally remove various medicines from the capsules.
The magnetic method of removing metal particles from the eye is widely used.
Most of us are familiar with the study of the work of the heart with the help of electrical sensors - an electrocardiogram. The electrical impulses produced by the heart create a magnetic field of the heart, which in max values is 10-6 of the Earth's magnetic field strength. The value of magnetocardiography is that it provides information about the electrically "silent" areas of the heart.
It should be noted that biologists are now asking physicists to give a theory of the primary mechanism of the biological action of the magnetic field, and physicists in response demand more verified biological facts from biologists. It is obvious that close cooperation of various specialists will be successful.
An important link uniting magnetobiological problems is the response of the nervous system to magnetic fields. It is the brain that first reacts to any changes in the external environment. It is the study of its reactions that will be the key to solving many problems of magnetobiology.
The simplest conclusion that can be drawn from the above is that there is no area of applied human activity where magnets would not be used.
References:
TSB, second edition, Moscow, 1957
Kholodov Yu. A. “Man in the magnetic web”, “Knowledge”, Moscow, 1972 Materials from the Internet encyclopedia
Putilov K. A. "Physics Course", "Physical Materials and Mathematics", Moscow, 1964.