What gas process occurs at constant pressure. Heat capacity. Thermodynamic processes with an ideal gas
Basic thermodynamic properties of ideal gases
In the study of thermodynamic processes, the equation of state is used
and the mathematical expression of the first law of thermodynamics
When studying the thermodynamic processes of ideal gases, in the general case, it is required to determine the equation of the process curve in PV , PT , VT diagram, establish a relationship between thermodynamic parameters and determine the following quantities:
− change internal energy working body
(the formula is valid not only for V = const, but also for any process)
− determine the external (thermodynamic) specific work
and available specific work
is the amount of heat involved in the thermodynamic process
Where is the heat capacity of the process
– change in enthalpy in a thermodynamic process
(the formula is valid not only for p = const, but also in any process)
- the proportion of heat that is spent on changing the internal energy in this process:
is the proportion of heat converted into useful work in this process
In the general case, any two of the three thermodynamic parameters ( P , V , T) can be changed arbitrarily. For practice, the following processes are of greatest interest:
Processes at constant volume (V = const) is isochoric.
At constant pressure ( P = const) is isobaric.
At constant temperature (T = const) is isothermal.
Process dq =0 (flowing without heat exchange of the working fluid with the environment) is an adiabatic process.
A polytropic process, which, under certain conditions, can be considered as generalizing in relation to all the main processes.
In the future, we will consider the 1st law of thermodynamics and the quantities included in it, as related to 1 kg of mass.
Process at constant volume
(isochoric process)
Such a process can be performed by a working fluid, for example, located in a vessel that does not change its volume, if heat is supplied to the working fluid from a heat source or heat is removed from the working fluid to the refrigerator.
In an isochoric process V = const and dV =0 . The isochoric process equation is obtained from the equation of state at V = const .
– Charles' law (*)
That is, at V = const gas pressure is proportional to absolute temperature. When heat is supplied, the pressure increases, and when heat is removed, it decreases.
Let's depict the process at V = const in pV , pT and VT diagrams.
AT p V - isochore diagram 1-2 - vertical straight line parallel to the axis p . In process 1-2, heat is supplied to the gas, the pressure increases, and consequently, from equation (*), the temperature increases. In the reverse process 2-1, heat is removed from the gas, as a result of which the internal energy of the gas decreases and its temperature decreases, i.e. process 1-2 - heating, 2-1 - gas cooling.
AT p T - isochore diagram - straight lines emerging from the origin with a slope (proportionality factor)
Moreover, the higher the volume level, the lower is the isochore.
In the VT - isochore diagram - straight lines parallel to the T axis.
External work of gas in isochoric process:
because the
Available specific work
The change in the internal energy of a gas in an isochoric process, if
The specific heat supplied to the working fluid, at
Since at V = const gas does no work dl =0 ), then the equation of the first law of thermodynamics will take the form:
That is, in the process V = const all the heat supplied to the working fluid is spent on increasing the internal energy, that is, on raising the temperature of the gas. When a gas is cooled, its internal energy decreases by the amount of heat removed.
The proportion of heat consumed to change the internal energy
The proportion of heat spent on doing work
Process at constant pressure
(isobaric process)
An isobaric process, for example, can take place in a cylinder under a piston that moves without friction so that the pressure in the cylinder remains constant.
With an isobaric process p = const , dp =0
The equation of the isobaric process is obtained when p = const from the equation of state:
– Gay-Lussac law (*)
In the process at p = const The volume of the gas is proportional to the temperature, that is, when the gas expands, the temperature, and hence the internal energy, increases, and when compressed, it decreases.
Let's depict the process in pV , pT , VT - diagrams.
AT pV-diagram of processes at p = const depicted as straight lines parallel to the axis V . The area of the rectangle 12 gives, on the appropriate scale, the work of the gas l. In process 1-2, heat is added to the gas as the specific volume increases, and hence, according to equation (*), the temperature increases. In the reverse process 2-1, heat is removed from the gas, as a result, the internal energy and temperature of the gas decrease, i.e. process 1-2 is heating, and 2-1 is cooling the gas.
AT VT- the isobar diagram is a straight line emerging from the origin of coordinates, with a slope .
AT pT- the isobar diagram is a straight line parallel to the axis T .
The work of a gas in an isobaric process ( p = const )
Since then
That is, if the temperature of the gas increases, then the work is positive.
Available job
because the ,.
The change in the internal energy of the gas, if
The amount of heat reported to the gas when heated (or given off by it when cooled), if
That is, the heat supplied to the working fluid in the isobaric process goes to increase its enthalpy, i.e. in an isobaric process is a total differential.
The equation of the first law of thermodynamics has the form
The fraction of heat consumed to change the internal energy in the isobaric process,
where k is the adiabatic index.
The proportion of heat consumed to perform work at p = const ,
In the MKT, n is the number of degrees of freedom.
For a monatomic gas n =3 and then φ=0.6, ψ=0.4, that is, 40% of the heat imparted to the gas goes to perform external work, and 60% goes to change the internal energy of the body.
For a diatomic gas n =5 and then φ=0.715, ψ=0.285, that is, ≈28.5% of the heat supplied to the gas goes to perform external work and 71.5% goes to change the internal energy.
For a triatomic gas n =6 and then φ=0.75, ψ=0.25, that is, 25% of the heat (steam engine) goes to perform external work.
Process at constant temperature
(isothermal process)
Such a thermodynamic process can take place in the cylinder of a reciprocating machine if, as heat is supplied to the working fluid, the piston of the machine moves, increasing the volume so much that the temperature of the working fluid remains constant.
At isothermal process T = const , dT =0.
From the equation of state
− Boyle-Mariotte law.
Therefore, in a process at constant temperature, the gas pressure is inversely proportional to the volume, i.e. With isothermal expansion, the pressure drops, and with compression, it increases.
We depict an isothermal process in pV , pT , VT − diagrams.
AT pV- diagram - the isothermal process is represented by an equilateral hyperbola, and the higher the temperature, the higher the isotherm is.
AT pT − diagram - isotherms - straight, parallel to the axis p .
AT VT − diagram - straight lines, parallel to the axis V .
dT =0, then
That is U = const , i = const – internal energy and enthalpy are not changed.
The equation of the first law of thermodynamics takes the form ( T = const)
That is, all the heat imparted to the gas in the isothermal process is spent on the work of expansion. In the reverse process, in the process of compression, heat is removed from the gas equal to external work compression.
Specific work in an isothermal process
Specific disposable work
From the last two equations it follows that in an isothermal process for an ideal gas, the available work is equal to the work of the process.
The heat imparted to the gas in process 1-2,
1st law of thermodynamics
It follows from this that when T = const l = l 0= q , those. work, work available, and the amount of heat received by the system are equal.
Because in an isothermal process dT =0, q = l = some final value, then from
we get that in the isothermal process C =∞. Therefore, to determine the amount of heat imparted to the gas in an isothermal process, using specific heat impossible.
The proportion of heat consumed to change the internal energy at T = const
and the proportion of heat expended on the performance of work,
Process without heat exchange with the environment
(adiabatic process)
In an adiabatic process, the energy exchange of the working fluid with the environment occurs only in the form of work. The working fluid is assumed to be thermally insulated from environment, i.e. there is no heat transfer between it and the environment;
q =0, and consequently dq =0
Then, the equation of the first law of thermodynamics will take the form
Thus, the change in internal energy and work in an adiabatic process are equivalent in magnitude and opposite in sign.
Therefore, work adiabatic process expansion occurs due to a decrease in the internal energy of the gas and, consequently, the temperature of the gas will decrease. The work of adiabatic compression goes entirely to increase the internal energy, i.e. to increase its temperature.
We obtain the adiabatic equation for an ideal gas. From the first law of thermodynamics
at dq =0 we get ( du = CV dT )
Heat capacity , where
Differentiating the equation of state pV = RT we get
Substituting RdT from (**) to (*)
or dividing by pV ,
Integrating at k = const , we get
The last equation is called the Poisson equation and is the adiabatic equation for .
It follows from the Poisson equation that
that is, during adiabatic expansion, the pressure decreases, and during compression, it increases.
We represent the isochoric process in pV , pT and VT - diagrams
Square V 1 12 V 2 under the adiabatic 1-2 on pV – diagram gives work l equal to the change in the internal energy of the gas
Comparing the adiabatic equation with the Boyle-Mariotte law ( T = const ) we can conclude that, since k >1, then, when expanding along the adiabat, the pressure drops more strongly than along the isotherm, i.e. in pV – the adiabatic diagram is larger than the isotherm, i.e. adiabat is an unequal hyperbola that does not intersect the coordinate axes.
We obtain the adiabatic equation in pT and VT − diagrams. In an adiabatic process, all three parameters change ( p , V , T ).
We get the relationship between T and V . Equations of state for points 1 and 2
whence dividing the second equation by the first
Substituting the pressure ratio from the Poisson adiabatic equation
or TVk -1= const is the adiabatic equation in VT - diagram.
Substituting into (*) (3) the ratio of volumes from the adiabatic equation (Poisson)
or − adiabatic equation in pT - chart. These equations are obtained under the assumption that k = const .
Work in an adiabatic process at CV = const
Considering the relationship between temperature T and V
Considering the ratio between T and p
Change in internal energy u=- l.
Available work, given that
,
Those. available work in k times more work of the adiabatic process l .
φ and ψ we do not find.
Polytropic process
A polytropic process is any arbitrary process that occurs at a constant heat capacity, i.e.
Then, the equation of the 1st law of thermodynamics will take the form
(*)
(1)
Thus, if C = const and CV = const , then the quantitative distribution of heat between internal energy and work in a polytropic process remains constant (for example, 1:2).
The proportion of heat consumed to change the internal energy of the working fluid
The proportion of heat spent on external work,
We obtain the equation of the polytropic process. To do this, we use the equation of the 1st law of thermodynamics (*)
From here, from (*) and (**)
Dividing the second equation (4) by the first (3)
We introduce a quantity called the polytropic exponent. Then,
Integrating this expression, we get
This equation is the polytropic equation in pV − chart. Potlitrope index n is constant for a particular process, and can vary from -∞ to +∞.
Using the equation of state, we can obtain the polytropic equation in VT and pT- diagrams.
From - polytropic equation in VT -
diagram.
From
− polytropic equation in pT - diagram.
The polytropic process is generalizing, and the main processes (isochoric, isothermal, adiabatic) are special cases of the polytropic process, each of which has its own value n . Thus, for each isochoric process n =±∞, isobaric n =0, isothermal n =1, adiabatic n = k .
Since the polytropic and adiabatic equations are the same in form and differ only in magnitude n(polytropic exponent instead of k − adiabatic exponent), we can write
operation of the polytropic process
disposable work of a polytropic process
Heat capacity of gas from , whence
Moreover, depending on n the heat capacity of the process can be positive, negative, equal to zero and varies from -∞ to +∞.
In C processes<0 всегда l> q those. to perform the work of expansion, in addition to the heat supplied, a part of the internal energy of the gas is consumed.
Change in the internal energy of a polytropic process
Heat imparted to gas in a polytropic process
Changing the enthalpy of the working fluid
Second law of thermodynamics
The first law of thermodynamics characterizes the processes of energy conversion from a quantitative point of view, i.e. he claims that heat can be converted into work, and work into heat, without establishing the conditions under which these transformations are possible. Thus, it only establishes the equivalence of various forms of energy.
The second law of thermodynamics establishes the direction and conditions of the process
As the first law of thermodynamics, the second law was derived from experimental data.
Experience shows that the conversion of heat into useful work can occur only when heat passes from a heated body to a cold one, i.e. when there is a temperature difference between the heat sink and the heat sink. It is possible to change the natural direction of heat transfer to the opposite only at the expense of the cost of work (for example, in refrigerators).
According to the 2nd law of thermodynamics
A process is impossible in which heat would transfer spontaneously from cold bodies to heated bodies.
Not all the heat received from the heat transfer can go into work, but only part of it. Part of the heat must go to the heat sink.
Thus, the creation of a device that, without compensation, would completely convert the heat of any source into work, and called perpetual motion machine of the second kind, impossible!
Reversible and irreversible processes
For any thermodynamic system, two states can be imagined, between which (Fig) two processes will occur: one from the first state to the second and the other vice versa, from the second state to the first.
The first process is called direct process, and the second reverse.
If a direct process is followed by a reverse process and the thermodynamic system returns to its original state, then such processes are considered to be reversible.
In reversible processes, the system in the reverse process passes through the same equilibrium states as in the direct process. In this case, neither in the environment nor in the system itself, there are any residual effects (no change in parameters, work performed, etc.). Through a direct process AB , and then reverse BA the final state of the system will be identical to the initial state.
The figure shows a mechanically reversible process setup. The installation consists of cylinder 1, piston 2 with table 3 and sand on it. Under the piston, the cylinder contains gas, which is under pressure from the sand on the table.
To create a reversible process, it is necessary to infinitely slowly remove one grain of sand after another. Then the process will be isothermal, and the pressure will be equal to the external pressure, and the system will be constantly in an equilibrium state. If the process is carried out in the opposite direction, i.e. infinitely slowly throw grains of sand on table 3, then the system will sequentially pass through the same equilibrium states and return to its original state (if there is no friction).
When expanding, the working body in a reversible process produces maximum work.
Brief theoretical part
The ratio of the heat δq received by a unit amount of a substance to the change in temperature dt is called the specific heat.
(1.1)Since the amount of heat δq depends on the nature of the process, the heat capacity of the system CX also depends on the conditions of the process.
The heat capacity, depending on the amount of substance, can be mass - C, volumetric - C "and molar µC. The relationship between them:

The physical meaning of the heat capacities of an ideal substance at V = const and P = const follows from the consideration of differential thermodynamic relations of the form:

After appropriate transformations, taking into account the properties of an ideal gas, we obtain:
(1.4)This indicates that the changes in internal energy and enthalpy are defined as:
(1.5)those. regardless of the nature of the process.
Relationships between CP and CV:

In accordance with the molecular kinetic theory of gases, the molar heat capacity at V = Const is proportional to the number of their degrees of freedom, expressed in Joule equivalent, and for one mole of gas is µСV = 3 × 4.19 = 12.5 J/(mol×K). Then, in accordance with Mayer's law, µСP = 5 × 4.19 = 20.8 J / (mol × K), which allows, depending on the atomicity of the gas and their degrees of freedom, to represent the values of molar heat capacities in the following form:
Table No. 1.1.
The heat capacity determined by equation (4.1) for given state parameters (P, v, T) is called true and can be expressed as:
CX = CX0 + ΔCX,(1.7)
where СX0 is the heat capacity of the gas in a discharged state (at P " 0) and depends only on temperature, and ΔСX determines the dependence of heat capacity on pressure and volume.
The average heat capacity CXm in the temperature range from T1 to T2 is expressed as:

If we accept that one of the limits, for example T1 = 273.15 K, then we can calculate the average heat capacities of gases in the temperature range from t1 = 0 °C to t2 = x °C and present their values in tabular form, see Appendix, Table No. 2 - No. 4.
The amount of heat transferred to the system according to equation (4.8) and using the heat capacity data, tables No. 2 - No. 4, taking into account (4.2), depending on the process, is calculated by the formulas:

For approximate calculations of the amount of heat at not very high temperatures we can take C = Const and then equations (1.14) taking into account (1.2) - (1.4) and the values of Table No. 4.1. will look like:

Task number 1-1. Air having a volume V = 15 m3 at a temperature t1 = 1500 °C and a pressure P = 760 mmHg is cooled isobarically to a temperature t2 = 250 °C. Determine the heat removed QP if: a) consider the heat capacity constant, b) use the formula µСP = 6.949 + + 0.000576×t.
Task number 1-2. The air flow is measured using an electric heater installed in the air duct. The air temperature before and after the heater is measured using two thermometers. Determine the hourly air flow G kg / h, if, when the electric heater with a power of 0.75 kW is turned on, the air temperature in front of the heater T1 = 288 K, and behind the heater T2 = 291.1 K. Also determine the air flow rate behind the heater, if its pressure is ( accepted by us unchanged) P = 870 mmHg, and the diameter of the air duct d = 90 mm.
Task number 1-3. As a result of the complete combustion of carbon in an atmosphere of pure oxygen, carbon dioxide CO2 was formed in the vessel at a pressure of P = 6.04 bar and a temperature of T1 = 1673 K. How much heat will be released when CO2 cools to a temperature of T2 = 293 K. Determine also what pressure will be established at the same time in the vessel and what pressure oxygen had in the vessel before combustion, if its temperature was 10 ° C. The volume of the vessel is taken unchanged and equal to 5 liters.
Task number 1-4. Find the amount of heat required to heat 1 Nm3 of a gas mixture of composition τ(CO2) = 14.5%; τ(O2) = 6.5%; τ(N2) = 79.0% from 200 to 1200 °C at P = Const and non-linear dependence of heat capacity on temperature.
Example. Air in the amount of 6 m3 at pressure P1 = 3 bar and temperature t1 = 25 °C is heated in the process P = Const to t2 = 130 °C. Determine the amount of heat supplied, considering C \u003d Const and C \u003d f (T).
QP \u003d m × CP × (t2 - t1) \u003d VH × C "P × (t2 - t1);
QP \u003d m × (CPm × t2 - CPm × t1) \u003d VH × (C "Pm × t2 - C" Pm × t1).
m = (P1 × V1 × µ) / (R × T1) = (3 × 105 × 6 × 2.896 × 10–2) / (8.314 × 298.15) = 21.03 kg.
VН = (Р1 × V1 × TN) / (РН × T1) = (3 × 105 × 6 × 273.15) / (101325 Pa × 298.15) = 16.28 nm3.
QP = 21.03 × (29.33/2.896 × 10–2) × (130 – 25) = 16.28 × (29.33/2.24 × 10–2) × (130 – 25) = 2236, 4 kJ.
QP = 21.03 kg × (1.0079 × 130 - 1.0042 × 25) = 16.28 × (1.3026 × 130 - 1.298 × 25) = 2227.5 kJ.
Discrepancy 0.40%.
Task number 1-5. A closed vessel with a capacity of V = 0.5 m5 contains carbon dioxide at P = 6 bar and T = 800 K. How will the gas pressure change if 100 kcal is subtracted from it? Accept dependence C = f(T) as linear.
Task number 1-6. A vessel with a capacity of 90 liters contains air at a pressure of 8 bar and a temperature of 303 K. Determine the amount of heat that must be imparted to the air in order to increase its pressure at V = Const to 16 bar. Accept dependence C = f(T) as non-linear. Give your answer in kcal.
Task number 1-7. What amount of heat must be expended to heat 2 m3 of air at a constant overpressure PM = 2 bar from t1 = 100 °C to t2 = 500 °C? how much work is done by the air? The air pressure on the barometer is taken equal to 760 mmHg.
Task number 1-8. Under isobaric heating from T1 = 313 K to T2 = 1023 K, a homogeneous gas does work l = 184 kJ/kg. Determine what kind of gas it is, how much heat is communicated to it, and how its pressure has changed in this case.
Task number 1-9. In the process of heat supply at constant pressure, the temperature of 0.9 nm3 of nitrogen rises from T1 = 288 K to T2 = 1873 K. Determine the changes in the enthalpy of nitrogen and the proportion of heat spent on increasing the internal energy.
Task number 1-10. The cylinder with a movable piston contains oxygen in the amount of VH = 0.3 nm3 at T1 = 318 K and P1 = 776 mmHg. A certain amount of heat is imparted to oxygen at P = Const, and then it is cooled to the initial temperature (318 K) at V = Const. Determine the amount of heat input, enthalpy change, internal energy and work done for both processes if it is known that at the end of isochoric cooling the oxygen pressure P3 = 0.588 bar. Draw the gas states in P-V and T-S coordinates.
Thermodynamic processes with an ideal gas.
Brief theoretical part
A thermodynamic process is understood as the interaction of the vehicle with the environment, as a result of which the vehicle is transferred from a certain initial state to a certain final state.
If the vehicle in which the process is running can be returned to the initial state so that during external environment no change occurs, the process is said to be reversible. If the initial state of the vehicle without changes in the external environment is irreversible, then the process is called irreversible.
Only reversible processes can be depicted graphically on state diagrams, since on them each point represents an equilibrium state.
The principle of conservation of energy, formulated by the first law of thermodynamics (formulas (2.1) - (2.3)), ultimately leads to an energy balance that relates the change in the energy reserve of the TS (internal energy) with the energy that passes the boundaries of the system when the process is completed in the form of work or heat .
A group of processes that, under certain conditions, generalizes for all processes and is characterized by a constant heat capacity is called polytropic.
For all processes, a general research method is established, which is as follows:
the equation of the process is derived;
establishes the relationship between the main parameters of the state of the vehicle;
the heat capacity of the process is determined;
· Changes of state functions are determined: internal energy, enthalpy, entropy;
process functions are calculated: heat and work;
· a graphical interpretation of thermodynamic processes in P – V and T – S coordinates is given.
The considered processes are considered to be reversible.
The main ratios according to points 1 - 5 are given in tables No. 2.1 - No. 2.3.
Table No. 2.1
Table No. 2.2
Table No. 2.3
The heat capacity in a polytropic process is:
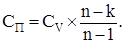
The figure below shows polytropic processes in P–V and T–S coordinates.

Example. Air having a volume V = 0.01 m3, at P1 = 10 bar and T1 = 298 K, expands in a cylinder with a movable piston to a pressure P2 = 1 bar. Determine the final volume, temperature, work of expansion, heat input, change in internal energy, enthalpy and entropy if expansion occurs: 1. isothermally; 2. adiabatically; 3. polytropic with polytropic index n = 1.3. Depict the process in P - V and T - S coordinates.
Isothermal expansion.
Volume at the end of expansion:
V2 = V1 × (P1/P2) = 0.01 × (10/1) = 0.1 m3.
Extension work:
L = P1 × V1 × ln(P1/P2) = 106 × 0.01 × ln(10/1) = 23 kJ.
QT = L = 23 kJ.
Since T1 = T2 = 298 K, then Δh = 0 and Δu = 0.
Entropy change:
ΔS \u003d Q / T \u003d 23/298 \u003d 0.07718 kJ / K.
adiabatic expansion.
Mass of gas in the cylinder:
m = (P1 × V1 × μ) / R × T1 = (106 × 0.01 × 2.896 × 10–2) / (8.314 × 298 K) = 0.117 kg.
Final volume:
V2 = V1 × (P1/P2) 1/k = 0.01 × (10/1) 1/1.4 = 0.0518 m3.
Air temperature at the end of the process:
T2 = T1 × (P2/P1) (k – 1) /k = 298 × (1/10) (1.4 – 1) /1.4 = 154.35 K.
The work of the gas during expansion:
L \u003d (P1 × V1 - P2 × V2) / (k - 1) \u003d (106 × 0.01 - 105 × 0.0518) / (1.4 - 1) \u003d 12 kJ.
Enthalpy:
Δh \u003d CP × (T2 - T1) \u003d 1.0189 × (154.35 - 298) \u003d - 146.36 kJ / kg;
ΔH \u003d m × Δh \u003d 0.117 × (- 146.36) \u003d - 17.12 kJ.
Internal Energy:
Δu \u003d CV × (T2 - T1) \u003d 0.7317 × (154.35 - 298) \u003d - 105.11 kJ / kg;
ΔU \u003d m × Δu \u003d 0.117 × (- 105.11) \u003d - 12.30 kJ.
When determining the change in state functions, due to a significant change in temperature in the process (298 - 154.35 = 146.65 K), we use the dependence of heat capacity on temperature C = f(T) (see Table No. 3 of the Appendix).
Polytropic extension with n = 1.3.
Final volume:
V2 = V1 × (P1/P2) 1/n = 0.01 × (10/1) 1/1.3 = 0.0588 m3.
Final temperature:
T2 = T1 × (V1/V2) n – 1 = 298 × (0.01/0.0588) 1.3 – 1 = 175.15 K.
The work of the gas during expansion:
L \u003d (P1 × V1 - P2 × V2) / (n - 1) \u003d (106 × 0.01 - 105 × 0.0588) / (1.3 - 1) \u003d 13.7 kJ.
The amount of heat supplied:
qP = CV × [(n – k) /(n – 1)] × (T2 – T1) = 0.7317 × [(1.3 – 1.4) /(1.3 – 1)] × (175 ,15 – – 298) = 29.96 kJ/kg;
QP = m × qP = 0.117 × 29.96 = 3.51 kJ.
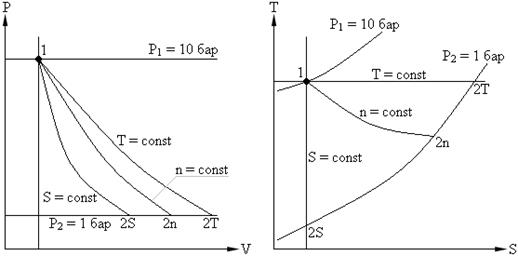
The change in the process was:
Enthalpy:
Δh \u003d CP × (T2 - T1) \u003d 1.0189 × (175.15 - 298) \u003d - 125.17 kJ / kg;
ΔH = m × Δh = 0.117 × (–125.17) = – 14.64 kJ.
Internal Energy:
Δu \u003d CV × (T2 - T1) \u003d 0.7317 × (175.15 - 298) \u003d - 89.89 kJ / kg;
ΔU = m × Δu = 0.117 × (–89.89) = – 10.52 kJ.
Tasks for independent solution.
Task number 2-1. In a closed room with a volume of V = 25 m3, there is air at a pressure of P1 = 730 mmHg and a temperature of T1 = 283 K. As a result of the heat supply, the pressure increased to P2 = 2.3 bar. Determine the amount of heat supplied QV, change in internal energy ΔU and enthalpy ΔH.
Task number 2-2.6. kg of nitrogen perform work LP = 343 kJ in the process of isobaric expansion. Determine the changes in the internal energy of nitrogen, if initial temperature its equal to T1 = 373 K.
Task number 2-3. Carbon monoxide is at an excess pressure of PM = 3.92 bar and occupies a volume of V = 5 m3, while the barometric pressure is equal to RB = 755 mmHg. Determine the change in internal energy and the amount of work expended if carbon monoxide is isobarically cooled from T1 = 573 K to T2 = 373 K.
Task number 2-4. How will the internal energy and enthalpy of 20 nm3 of oxygen change during isobaric heating from 373 K to 1173 K if the pressure P = 9.8 bar. What is the work done by the gas?
Task No. 2-5.0.6 nm3 of air with isobaric heat supply does work LP = 15.68 kJ. Determine the temperature T2 and the volume of air V, if in the initial state its temperature and pressure were, respectively, P1 = 4.42 bar and T1 = 293 K.
Task number 2-6. Oxygen at a temperature T1 = 353 K and a pressure PB equal to 320 mmHg is compressed at T = Const to an overpressure PM = 12 bar. How many times does the volume of oxygen decrease if the barometric pressure РБ = 745 mmHg?
Task number 2-7. 10 kg of oxygen expand at T = 423 K = Const from the initial pressure P1 = 14.7 bar and produce work LT = 2969.4 kJ. Determine the pressure at the end of the expansion and depict the process in P - V and T - S coordinates.
Task number 2-8. A cylinder with a movable piston contains 3.5 m3 of nitrogen at a pressure P1 = 1.47 bar. During isothermal compression, 461 kJ of heat is removed. Determine pressure P2 and volume V2 of nitrogen at the end of compression.
Task number 2-9. 0.4 kg of air at T1 = 573 K and P1 = 1.98 bar expand isothermally to V2 = 1.68 m3/kg, and then are compressed isobarically and, finally, by isochoric heating, again return to their original state. Determine for each process ΔH, ΔS, ΔU, as well as heat and work L. Also determine the parameters (P, v, T) for all points and depict the processes in P - V and T - S coordinates.
Task number 2-10. 0.3 Nm3 of air is isothermally compressed from the initial state P1 = 7.35 bar, T1 = 573K to some final state P2, V2. Determine the values of P2 and V2 if it is known that 167.6 kJ of heat was removed during the isothermal compression. Determine also the change in internal energy and enthalpy of air.
Task number 2-11. 1. Nm3 of air expands adiabatically from the initial state 1 (P1 = 6 atm, t1 = 300 °C) to the state where V2 = 3V3; then it is compressed isothermally to the initial value of the specific volume V3 = V1. Determine the parameters (P, v, T) of points 1, 2 and 3 and the total work done by the gas. Represent the process in P - V and T - S coordinates.
Task number 2-12. The work spent on the adiabatic compression of 3 kg of air is (- 471) kJ. The initial state of the air is characterized by the parameters T1 = 288 K and P1 = 1 bar. Determine the final temperature and the change in internal energy.
Task number 2-13. 1. kg of air at pressure P1 = 4 bar and temperature T1 = 373 K expands to pressure P2 = 1 bar. Determine the final temperature, the amount of heat and the work done if the expansion occurs: a) isochorically, b) isothermally, c) adiabatically and d) polytropically with exponent n = 1.2. Depict the process in P - V and T - S coordinates.
Problem #2-14. A cylinder with a capacity of 100 liters contains air at a pressure P1 = 50 bar and a temperature T1 = 293 K. The ambient pressure P2 = 1 bar. Determine the useful work that can be done by air when it expands to ambient pressure along the isotherm and adiabatically, as well as the final air temperature in the balloon after adiabatic expansion.
Task number 2-15. 1 kg of air at a temperature T1 = 290 K is compressed adiabatically to a volume that is 1/5 of the initial volume, and then expands isothermally to the initial volume. Determine the work done by the air as a result of both processes. Depict the process in P - V and T - S coordinates.
Problem #2-16. With polytropic expansion of 1 kilomole of gas, its volume increased by 20%, and absolute temperature decreased by 12%. Determine the polytropic index, the value of work lP kJ/mol, if T1 = 490 K.
Problem #2-17. 50 kJ/kg of heat is supplied to 1 kg of air during its compression in the polytropic process. Determine the polytropic index, the change in internal energy and the work of compression if the air temperature increased by 100 K during the process.
Problem number 2-18. 1 kg of nitrogen in the initial state has parameters P1 = 25 bar and T1 = 973 K. After polytropic expansion (n = 1.18), the nitrogen pressure becomes equal to P2 = 105 N/m2. Determine ΔU, ΔН in the process, as well as the amount of heat qP and the work of expansion lP.
1. A process that occurs at a constant volume is called ___ isochoric ________
2. A process occurring at constant pressure is called ___ isobaric ______
3. A process that takes place at a constant temperature is called ___ isothermal ___
4. A process that proceeds without heat exchange with the external environment is called _______________adiabatic ___________________________
5. A generalizing thermodynamic process, in which the proportion of heat used to change the internal energy of the system remains constant, is called _________polytropic______________
6. The amount of heat that needs to be imparted to a body in order to change its temperature by one kelvin or degree Celsius is called ____ heat capacity _______
7. For a heat engine cycle, the ratio of work done per cycle to the amount of heat input is ______ cycle efficiency ______
8. The amount of heat required to convert 1 kg of a liquid heated to the boiling point at constant pressure into dry saturated steam is called ________________ heat of vaporization ____________
9. The molecular method of heat transfer in a continuous medium in the presence of a temperature gradient is called ____________ thermal conductivity ______________________________
10. Heat transfer, carried out by the combined action of heat conduction and convection, is called ___________ convective heat transfer ___________________________
11. The amount of heat transferred through any surface per unit time is called ____________ heat flux __________
12. The heat flux per unit area of the surface through which it passes is called _____ heat flux_density __________________________
13. The parameters of the state of a thermodynamic system are
15. A system that can exchange with the environment and matter and energy is called
1) open |
3) adiabatic |
2) closed |
4) closed |
16. A system that can exchange energy but not matter with the environment is called
18. The equation of state for an arbitrary amount of ideal gas has the form
The following designations are accepted in the answer options:
p - pressure
v- specific volume
m is the mass of gas
T - thermodynamic temperature
t- temperature in Celsius
M is the number of kilomoles of gas
R- gas constant
Universal gas constant
19. Mutual transformation occurs in thermomechanical systems
20. A process without heat exchange with the environment is called
21. A process that takes place at a constant temperature is called
1) isochoric |
4) adiabatic |
2) isobaric |
5) isentropic |
3) isothermal |
22. A process that takes place at a constant volume is called
24. The factor that has the greatest influence on the specific heat capacity of a gas is
25. The conditional fuel is called
1) the fuel most suitable for specific conditions of use
2) medium quality coal
3) fuel with a lower calorific value of 29.31 MJ / kg
4) fuel with a lower calorific value of 35.0 MJ/kg
26. The figures show installation diagrams
1) a) steam power; b) gas turbine; c) vapor compressor refrigeration
2) a) vapor compressor refrigeration; b) gas turbine; c) steam power
3) a) absorption refrigeration; b) gas turbine; c) steam power
4) a) gas turbine; b) vapor compressor refrigeration; c) steam power
27. A heat pump is
1) a refrigerating machine used for heat supply (heating) purposes
2) a pump of any design used for pumping liquid
coolants
3) a device for transferring heat from a "hot" source to a "cold" one
4) a device for pumping temperature from one heat source to
28. The heat flux density during heat transfer is calculated by the formula
1)
|
3)
|
2)
|
4)
|
The following notations are used in the given answer options:
-coefficient of thermal conductivity
-heat transfer coefficient
– heat transfer coefficient
– wall thickness
and
– temperature of heat carriers
and
are the temperatures of the wall surfaces
Match the answers |
29. In the Otto cycle shown in the diagrams
notation processes
1) 1-2 A) isochoric heat supply
2) 2-3 B) isobaric heat supply
3) 3-4 V) isochoric heat removal
4) 4-1 D) isobaric heat removal
D) isothermal heat supply
E) isothermal heat removal
G) adiabatic expansion
H) adiabatic compression
1.__z___; 2.__a___; 3.__f___; 4.__in___